Explain How Work Is Related To Energy
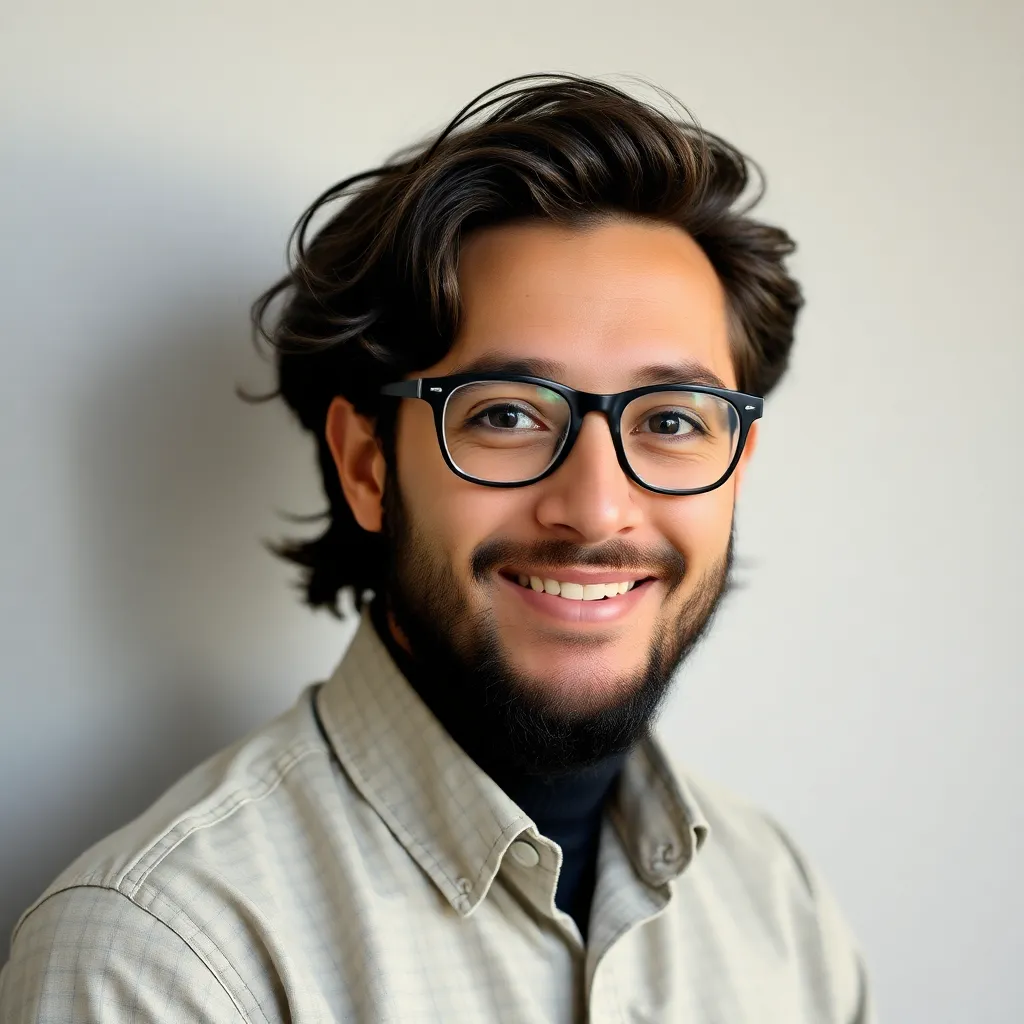
listenit
Apr 24, 2025 · 7 min read

Table of Contents
How Work is Related to Energy: A Deep Dive into Physics and Everyday Life
Work and energy are fundamental concepts in physics that are deeply intertwined. Understanding their relationship is crucial to comprehending how the world around us functions, from the simple act of lifting an object to the complexities of power generation and technological advancements. This article delves into the precise definition of work in physics, explores the different forms of energy, and meticulously explains their interconnectedness, providing illustrative examples from everyday life and advanced applications.
Defining Work in Physics: More Than Just Effort
In everyday language, "work" often refers to any physical or mental exertion. However, in physics, work has a much more specific definition. Work is done when a force causes an object to move in the direction of the force. This is crucial; simply applying a force doesn't constitute work unless there's displacement in the direction of the force.
Consider these scenarios:
- Scenario 1: You push against a wall with all your might. You exert a significant force, but the wall doesn't move. No work is done because there's no displacement.
- Scenario 2: You lift a box from the floor to a table. You apply an upward force, and the box moves upward. Work is done because a force caused a displacement in the direction of the force.
The formula for calculating work is:
W = Fd cos θ
Where:
- W represents work (measured in Joules, J)
- F represents the force applied (measured in Newtons, N)
- d represents the displacement (measured in meters, m)
- θ represents the angle between the force and the displacement.
This formula highlights the importance of the direction of the force relative to the displacement. If the force is applied at an angle to the displacement (θ ≠ 0°), only the component of the force in the direction of the displacement contributes to the work done. If the force is perpendicular to the displacement (θ = 90°), no work is done.
Examples of Work in Everyday Life:
- Lifting weights: When you lift weights, you apply an upward force, and the weights move upward. The work done is equal to the weight of the weights multiplied by the height they are lifted.
- Pushing a shopping cart: Pushing a shopping cart across the supermarket floor involves applying a force to overcome friction and move the cart. The work done is the force applied multiplied by the distance the cart is moved.
- Cycling uphill: Cycling uphill involves applying force to overcome gravity and move the bicycle uphill. The work done is the force exerted multiplied by the distance climbed.
The Inseparable Link: Work and Energy
The fundamental principle underlying the relationship between work and energy is the work-energy theorem. This theorem states that the net work done on an object is equal to the change in its kinetic energy. Kinetic energy is the energy an object possesses due to its motion.
Mathematically, the work-energy theorem is expressed as:
W<sub>net</sub> = ΔKE
Where:
- W<sub>net</sub> represents the net work done on the object.
- ΔKE represents the change in the object's kinetic energy (KE<sub>final</sub> - KE<sub>initial</sub>).
This equation signifies that work is a mechanism for transferring or transforming energy. When work is done on an object, its energy changes. This change can manifest as an increase in kinetic energy (faster movement), a change in potential energy (higher position), or a change in other forms of energy.
Different Forms of Energy and Their Relationship to Work
Energy exists in numerous forms, and each is related to work in different ways. Here are some key examples:
1. Kinetic Energy:
As mentioned earlier, kinetic energy is the energy of motion. The work done on an object can increase its kinetic energy, causing it to move faster. Conversely, when an object slows down, it does work on its surroundings, resulting in a decrease in its kinetic energy.
The formula for kinetic energy is:
KE = ½mv²
Where:
- KE represents kinetic energy (measured in Joules, J)
- m represents the object's mass (measured in kilograms, kg)
- v represents the object's velocity (measured in meters per second, m/s)
2. Potential Energy:
Potential energy is stored energy due to an object's position or configuration. There are different types of potential energy:
-
Gravitational Potential Energy: This is the energy stored due to an object's height above a reference point. Work is done against gravity to lift an object, increasing its gravitational potential energy. When the object falls, its potential energy is converted into kinetic energy. The formula is:
PE<sub>g</sub> = mgh
Where:
- PE<sub>g</sub> represents gravitational potential energy (J)
- m represents mass (kg)
- g represents acceleration due to gravity (approximately 9.8 m/s²)
- h represents height (m)
-
Elastic Potential Energy: This is the energy stored in a deformed elastic object, like a stretched spring or a compressed rubber band. Work is done to deform the object, storing energy as elastic potential energy. When the object returns to its original shape, this stored energy is released. The formula is:
PE<sub>e</sub> = ½kx²
Where:
- PE<sub>e</sub> represents elastic potential energy (J)
- k represents the spring constant (N/m)
- x represents the displacement from equilibrium (m)
3. Thermal Energy (Heat):
Thermal energy is the energy associated with the random motion of particles within a substance. Friction is a common mechanism for converting mechanical work into thermal energy. When two surfaces rub against each other, work is done against friction, generating heat. This heat increases the thermal energy of the objects involved.
4. Chemical Energy:
Chemical energy is stored in the bonds between atoms and molecules. When chemical reactions occur, this energy can be released or absorbed. For example, the combustion of fuel in an engine converts chemical energy into mechanical work. The process of photosynthesis utilizes light energy to perform work and store chemical energy in plant tissues.
5. Electrical Energy:
Electrical energy is associated with the movement of electric charges. Work is done to move charges through a potential difference, resulting in electrical energy. This energy can be used to power various devices, converting it into other forms of energy like light, heat, or mechanical energy.
Work and Energy in Advanced Applications
The principles of work and energy are not just limited to simple scenarios; they are fundamental to various advanced technologies and scientific fields:
- Power Generation: Power plants, whether based on fossil fuels, nuclear reactions, or renewable sources, are all essentially about converting one form of energy (chemical, nuclear, kinetic) into mechanical work, which then generates electricity.
- Rocket Propulsion: Rockets use the principle of conservation of momentum. The combustion of fuel generates hot gases that are expelled at high velocity, performing work on the gases. In accordance with Newton's third law, an equal and opposite force propels the rocket upward.
- Mechanical Engineering: Designers of machines and structures constantly consider the interplay of work and energy to optimize efficiency and performance.
- Biomechanics: Studying human and animal movement involves analyzing the work done by muscles and the energy transformations involved in locomotion and other physical activities.
Conclusion: The Ubiquitous Role of Work and Energy
Work and energy are inextricably linked concepts that are crucial for understanding the physical world. Work is the mechanism by which energy is transferred or transformed. Understanding their relationship allows us to analyze a vast array of phenomena, from simple everyday actions to complex technological advancements and scientific discoveries. From the energy stored in food that fuels our bodies to the power that lights our homes, work and energy are the fundamental driving forces behind it all. The principles governing work and energy are not just abstract concepts but rather the very foundation of how our universe functions and how we interact with it. A deeper understanding of these principles empowers us to design more efficient machines, develop sustainable technologies, and improve our understanding of the natural world.
Latest Posts
Latest Posts
-
A Row In The Periodic Table
Apr 25, 2025
-
What Is The Greatest Common Factor Of 75 And 90
Apr 25, 2025
-
Valence Bond Theory And Molecular Orbital Theory
Apr 25, 2025
-
Nitrogen Number Of Protons Neutrons And Electrons
Apr 25, 2025
-
Least Common Multiple Of 9 And 16
Apr 25, 2025
Related Post
Thank you for visiting our website which covers about Explain How Work Is Related To Energy . We hope the information provided has been useful to you. Feel free to contact us if you have any questions or need further assistance. See you next time and don't miss to bookmark.