Draw The Product Of The Complete Hydrogenation Of Ethyne.
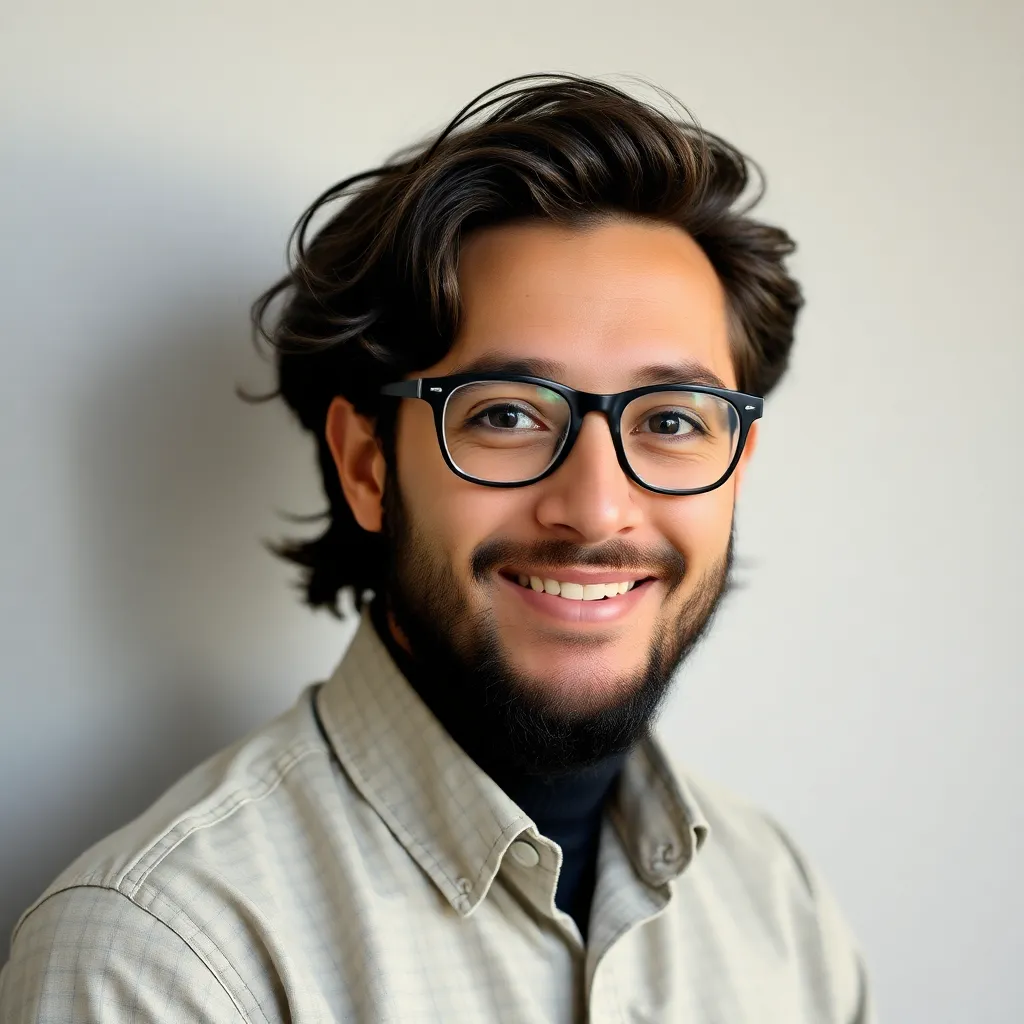
listenit
Apr 27, 2025 · 6 min read

Table of Contents
Draw the Product of the Complete Hydrogenation of Ethyne: A Deep Dive into Alkene and Alkane Chemistry
The complete hydrogenation of ethyne (also known as acetylene) is a fundamental reaction in organic chemistry, illustrating the crucial concept of reduction and the transformation of unsaturated hydrocarbons into saturated ones. This process involves the addition of hydrogen atoms across the carbon-carbon triple bond, ultimately yielding a saturated alkane. This article will delve into the details of this reaction, exploring the mechanism, the product formed, and its implications within broader organic chemistry principles. We’ll also explore related concepts and practical applications.
Understanding Ethyne and its Reactivity
Ethyne, with its chemical formula C₂H₂, is the simplest alkyne. Its structure features a carbon-carbon triple bond, characterized by the presence of one sigma (σ) bond and two pi (π) bonds. This triple bond is responsible for ethyne's high reactivity. The pi bonds, being less stable than sigma bonds, are susceptible to addition reactions, where atoms or groups are added across the multiple bond. Hydrogenation is a prime example of such an addition reaction.
The Triple Bond's Unique Properties
The triple bond significantly impacts the molecule's geometry and reactivity. The carbon atoms in ethyne exhibit sp hybridization, resulting in a linear molecular geometry. This linear arrangement contributes to the molecule's unique reactivity, making it readily susceptible to reactions that saturate the triple bond.
The Hydrogenation Process: A Step-by-Step Explanation
The complete hydrogenation of ethyne involves the addition of two molecules of hydrogen (H₂) across the triple bond. This reaction doesn't occur spontaneously under standard conditions; it requires a catalyst to facilitate the process. Common catalysts used include platinum (Pt), palladium (Pd), and nickel (Ni). These transition metals provide a surface where the hydrogen molecules can adsorb and dissociate, enabling interaction with the ethyne molecule.
Step 1: Addition of the First Hydrogen Molecule
The first hydrogen molecule adds to the ethyne molecule, breaking one of the pi bonds. This results in the formation of ethene (ethylene), an alkene containing a carbon-carbon double bond. This intermediate step is crucial to understanding the overall transformation. The reaction can be represented as follows:
C₂H₂ + H₂ → C₂H₄
This reaction is exothermic, meaning it releases heat. The heat released contributes to the overall thermodynamic favorability of the process.
Step 2: Addition of the Second Hydrogen Molecule
The second hydrogen molecule then adds to the ethene molecule, breaking the remaining pi bond and forming ethane, a saturated alkane. The reaction can be represented as:
C₂H₄ + H₂ → C₂H₆
This step, like the first, is also exothermic.
The Catalyst's Role: A Closer Look
The catalyst plays a vital role in the hydrogenation process. It lowers the activation energy of the reaction, allowing it to proceed at a reasonable rate. Without the catalyst, the reaction would be extremely slow, if it occurred at all. The catalyst facilitates the adsorption of both hydrogen and ethyne onto its surface, bringing them into close proximity and promoting the interaction necessary for bond formation.
Drawing the Product: Ethane (C₂H₆)
The final product of the complete hydrogenation of ethyne is ethane, a simple alkane with the formula C₂H₆. Its structure is represented as:
H H
| |
H - C - C - H
| |
H H
This structure shows a single carbon-carbon bond (sigma bond) and each carbon atom bonded to three hydrogen atoms. Ethane is a saturated hydrocarbon, meaning it contains only single bonds and no multiple bonds. Its geometry is tetrahedral around each carbon atom, with bond angles of approximately 109.5°.
Comparing Ethyne, Ethene, and Ethane
The hydrogenation process showcases the gradual saturation of carbon-carbon bonds. Comparing the three compounds - ethyne, ethene, and ethane - highlights the changes in properties associated with the degree of unsaturation:
Compound | Formula | Bond Type | Geometry | Reactivity |
---|---|---|---|---|
Ethyne | C₂H₂ | Triple bond | Linear | Highly reactive |
Ethene | C₂H₄ | Double bond | Trigonal planar | Moderately reactive |
Ethane | C₂H₆ | Single bond | Tetrahedral | Relatively unreactive |
This table clearly illustrates how the presence of multiple bonds increases reactivity. Ethyne, with its triple bond, is the most reactive, followed by ethene with its double bond. Ethane, with only single bonds, is the least reactive.
Practical Applications and Significance
The hydrogenation of alkynes, including ethyne, has several important industrial applications:
-
Production of Alkanes: This process is crucial for the production of alkanes, which are valuable components of fuels and other petrochemicals. The conversion of readily available alkynes into alkanes allows for efficient utilization of resources.
-
Synthesis of other Chemicals: The hydrogenated alkenes and alkanes can serve as precursors for the synthesis of numerous other organic chemicals, demonstrating the importance of this reaction in chemical synthesis.
-
Polymer Chemistry: The hydrogenation of unsaturated polymers is often used to modify their properties, such as increasing their stability and durability.
-
Food Industry: Hydrogenation is also used in the food industry to produce saturated fats from unsaturated fats, although this practice has become less common due to health concerns.
Further Exploration: Partial Hydrogenation and Regioselectivity
It's important to note that the complete hydrogenation described above leads to the formation of a single product, ethane. However, under controlled conditions, partial hydrogenation can also occur, stopping at the alkene stage (ethene). This controlled reaction requires careful adjustment of the reaction conditions, such as catalyst choice and reaction temperature and pressure.
Furthermore, with more complex alkynes, regioselectivity and stereoselectivity can become important considerations. Regioselectivity refers to the preference for the addition of hydrogen to one side of the triple bond over another. Stereoselectivity refers to the preference for forming one stereoisomer over another. These considerations become particularly relevant when dealing with substituted alkynes.
Conclusion: A Cornerstone of Organic Chemistry
The complete hydrogenation of ethyne to ethane is a fundamental reaction illustrating the addition of hydrogen to unsaturated hydrocarbons. Understanding this process is crucial for grasping the principles of alkane and alkene chemistry, catalysis, and the practical applications of these reactions in various industries. By exploring the mechanism, the role of catalysts, and the properties of the products, we gain a deeper understanding of this important organic chemistry transformation. The ability to control this reaction, either towards complete or partial hydrogenation, further expands its versatility and importance in chemical synthesis and industrial processes. This thorough understanding serves as a building block for tackling more complex organic reactions and further expanding our knowledge in the field.
Latest Posts
Latest Posts
-
Does Aluminum Have A Fixed Charge
Apr 28, 2025
-
Dissolving Of Salt In Water Physical Or Chemical
Apr 28, 2025
-
How To Find The Point Estimate In Statistics
Apr 28, 2025
-
Find The Equation Of The Perpendicular Bisector Of Ab
Apr 28, 2025
-
What Landform Is Created By A Divergent Plate Boundary
Apr 28, 2025
Related Post
Thank you for visiting our website which covers about Draw The Product Of The Complete Hydrogenation Of Ethyne. . We hope the information provided has been useful to you. Feel free to contact us if you have any questions or need further assistance. See you next time and don't miss to bookmark.