Draw All Resonance Structures For The Carbonate Ion Co32-
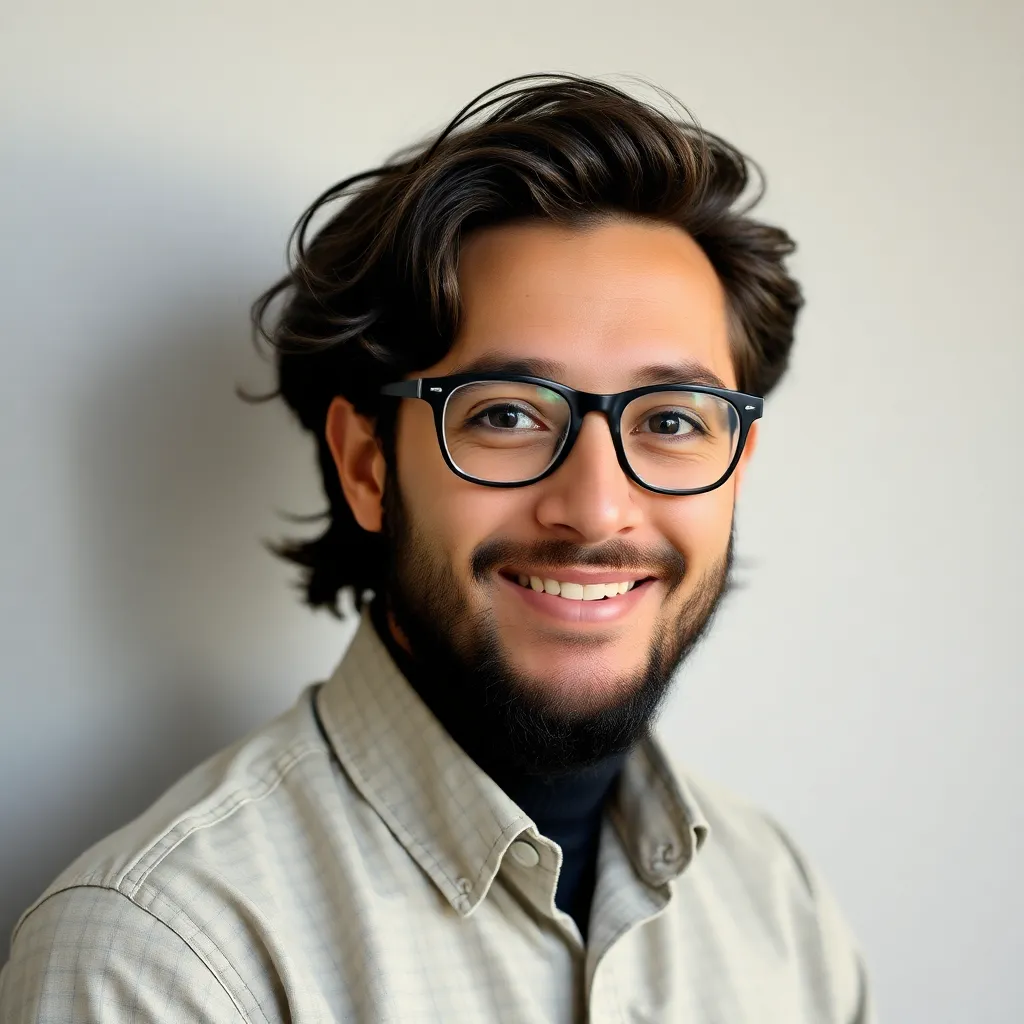
listenit
May 10, 2025 · 6 min read
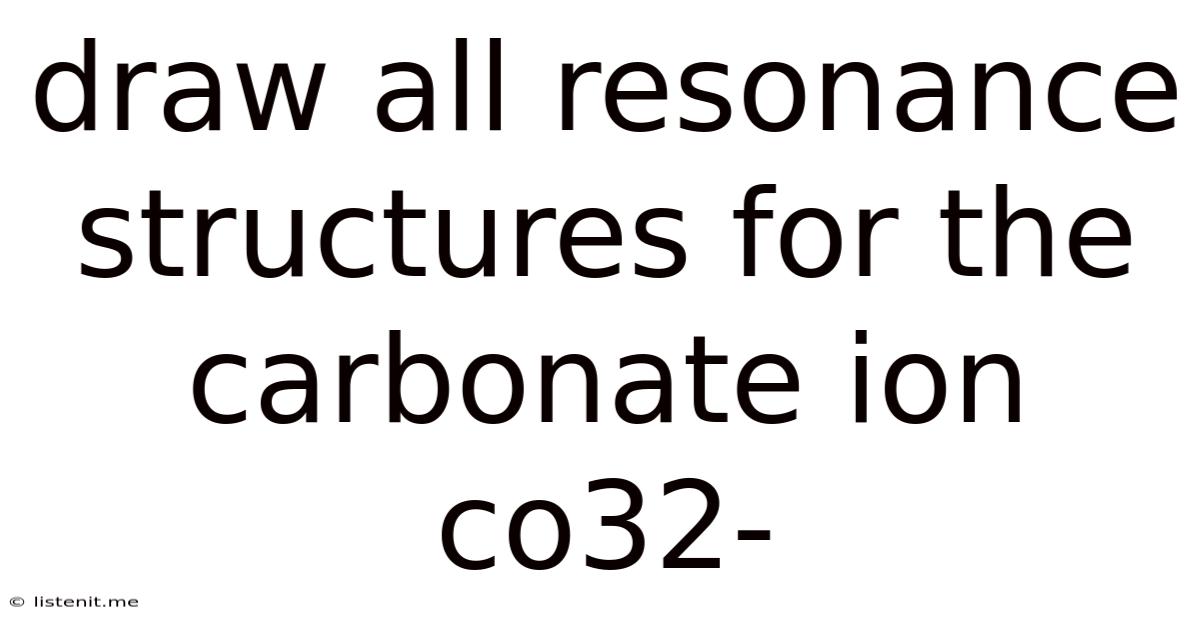
Table of Contents
Drawing All Resonance Structures for the Carbonate Ion (CO₃²⁻)
The carbonate ion, CO₃²⁻, is a classic example in chemistry illustrating the concept of resonance. Understanding its resonance structures is crucial for grasping its bonding, geometry, and reactivity. This article will delve deep into the process of drawing all resonance structures for the carbonate ion, explaining the underlying principles and providing a step-by-step guide. We'll also explore the implications of resonance for the properties of the carbonate ion.
Understanding Resonance Structures
Before we begin drawing the resonance structures, let's clarify what resonance structures are and why they're important. Resonance structures are multiple Lewis structures that can be drawn for a single molecule or ion, where the only difference between them is the placement of electrons (specifically, pi electrons and lone pairs). No single resonance structure accurately represents the true bonding in a molecule or ion exhibiting resonance. Instead, the actual molecule is a hybrid – a weighted average – of all the contributing resonance structures.
The carbonate ion is a prime example because the double bond can be located between the carbon atom and any of the three oxygen atoms. This means we'll have multiple Lewis structures, each contributing to the overall picture of the carbonate ion's bonding.
Step-by-Step Guide to Drawing Resonance Structures of CO₃²⁻
Let's follow these steps to draw all possible resonance structures of the carbonate ion:
Step 1: Count Valence Electrons
First, we need to determine the total number of valence electrons in the carbonate ion. Carbon has 4 valence electrons, each oxygen atom has 6, and we add two more electrons due to the 2- charge:
4 (C) + 3 * 6 (O) + 2 (charge) = 24 valence electrons
Step 2: Draw a Skeletal Structure
Next, we create a skeletal structure. Carbon is the central atom, with the three oxygen atoms surrounding it:
O
/ \
O O
\ /
C
Step 3: Connect Atoms with Single Bonds
Connect each oxygen atom to the carbon atom with a single bond. Each single bond uses two electrons, so we've used 6 electrons (3 bonds * 2 electrons/bond).
Step 4: Distribute Remaining Electrons as Lone Pairs
We have 18 electrons left (24 total - 6 used). We distribute these electrons as lone pairs around the oxygen atoms, giving each oxygen atom three lone pairs (6 electrons). This completes the octets for all oxygen atoms.
O
/ \
O O
\ /
C
Step 5: Check for Octet Rule Satisfaction
At this point, carbon only has six electrons (making it electron deficient). To satisfy the octet rule for carbon, we need to form a double bond with one of the oxygen atoms. This is where the resonance comes in.
Step 6: Draw Resonance Structures
Since we can form the double bond with any of the three oxygen atoms, we have three equivalent resonance structures. Here's how they look:
Resonance Structure 1:
O
/ \\
O O
\ /
C=O
Resonance Structure 2:
O
/ \\
O=C O
\ /
O
Resonance Structure 3:
O=C
/ \
O O
\ /
O
Each structure shows a double bond between carbon and one oxygen atom, and single bonds between carbon and the other two oxygen atoms. The formal charges need to be considered to ensure these structures are valid contributors to the resonance hybrid.
Formal Charge Calculation and its Significance
Calculating formal charges helps verify the validity and relative contribution of each resonance structure. The formal charge is calculated as:
Formal Charge = (Valence Electrons) - (Non-bonding Electrons) - (1/2 * Bonding Electrons)
Let's calculate the formal charges for each atom in Resonance Structure 1:
- Carbon: 4 - 0 - (1/2 * 8) = 0
- Double-bonded Oxygen: 6 - 4 - (1/2 * 4) = 0
- Single-bonded Oxygens: 6 - 6 - (1/2 * 2) = -1 (each)
Notice that the sum of formal charges equals -2, which matches the overall charge of the carbonate ion. The formal charges in the other two resonance structures will be the same, just with the zero and -1 charges distributed among different oxygen atoms. The fact that the negative charges reside on the electronegative oxygen atoms is favorable, making these resonance structures significant contributors.
The Resonance Hybrid
It's crucial to understand that none of the individual resonance structures accurately represent the carbonate ion. The actual carbonate ion is a resonance hybrid, a blend of all three structures. The double bond is delocalized across all three C-O bonds; the bond order for each C-O bond is 1.33 (average of one double bond and two single bonds). This delocalization of electrons significantly impacts the properties of the carbonate ion.
Properties Influenced by Resonance
The resonance stabilization in the carbonate ion leads to several important consequences:
-
Increased Stability: The delocalized electrons create a more stable ion than if the double bond were localized between one carbon-oxygen pair. This increased stability is reflected in the carbonate ion's lower reactivity compared to similar ions without resonance.
-
Equal Bond Lengths: The three C-O bonds are experimentally found to be identical in length, intermediate between a typical single and double bond. This experimental observation is direct evidence for resonance and the delocalized nature of the pi electrons.
-
Planar Geometry: The carbonate ion adopts a trigonal planar geometry, with the carbon atom at the center and the three oxygen atoms arranged symmetrically around it in a plane. This geometry is consistent with sp² hybridization of the carbon atom and the delocalized pi system.
-
Reactivity: The delocalized electron system makes the carbonate ion less reactive towards electrophilic attack compared to a similar molecule without resonance stabilization.
Applications and Importance of Carbonate Ion
The carbonate ion is incredibly prevalent in chemistry and biology, playing a crucial role in various processes and applications.
-
Inorganic Chemistry: Carbonate salts are widely used in various industrial applications, such as in cement production, glass manufacturing, and water treatment.
-
Biochemistry: The carbonate ion is vital in biological systems, acting as a buffer in blood, crucial for maintaining blood pH within a narrow range. It also plays a significant role in the formation of shells and skeletons in marine organisms.
-
Environmental Science: The carbonate-bicarbonate buffer system is essential for regulating the pH of natural water bodies. Understanding carbonate chemistry is critical in climate change research due to the impact of CO2 dissolution in the oceans and the formation of carbonic acid.
-
Material Science: Carbonate-based materials are being explored for various applications, including in batteries and catalysts.
Conclusion
The carbonate ion (CO₃²⁻) provides a powerful illustration of resonance and its impact on molecular properties. Drawing all its resonance structures, understanding formal charges, and appreciating the resonance hybrid are essential for grasping the ion's stability, geometry, and reactivity. Its widespread presence in both inorganic and biological systems highlights its importance across multiple scientific disciplines. By understanding the concept of resonance applied to the carbonate ion, we can gain a deeper appreciation for the complex world of chemical bonding and molecular structure.
Latest Posts
Latest Posts
-
The Standard Unit Of Mass Is
May 11, 2025
-
What Is The Value Of 81
May 11, 2025
-
How To Find Density Of A Cube
May 11, 2025
-
What Is The Oxidation Number Of Cr In K2cr2o7
May 11, 2025
-
How Do You Solve For X And Y
May 11, 2025
Related Post
Thank you for visiting our website which covers about Draw All Resonance Structures For The Carbonate Ion Co32- . We hope the information provided has been useful to you. Feel free to contact us if you have any questions or need further assistance. See you next time and don't miss to bookmark.