Do Smaller Molecules Have More Microstates
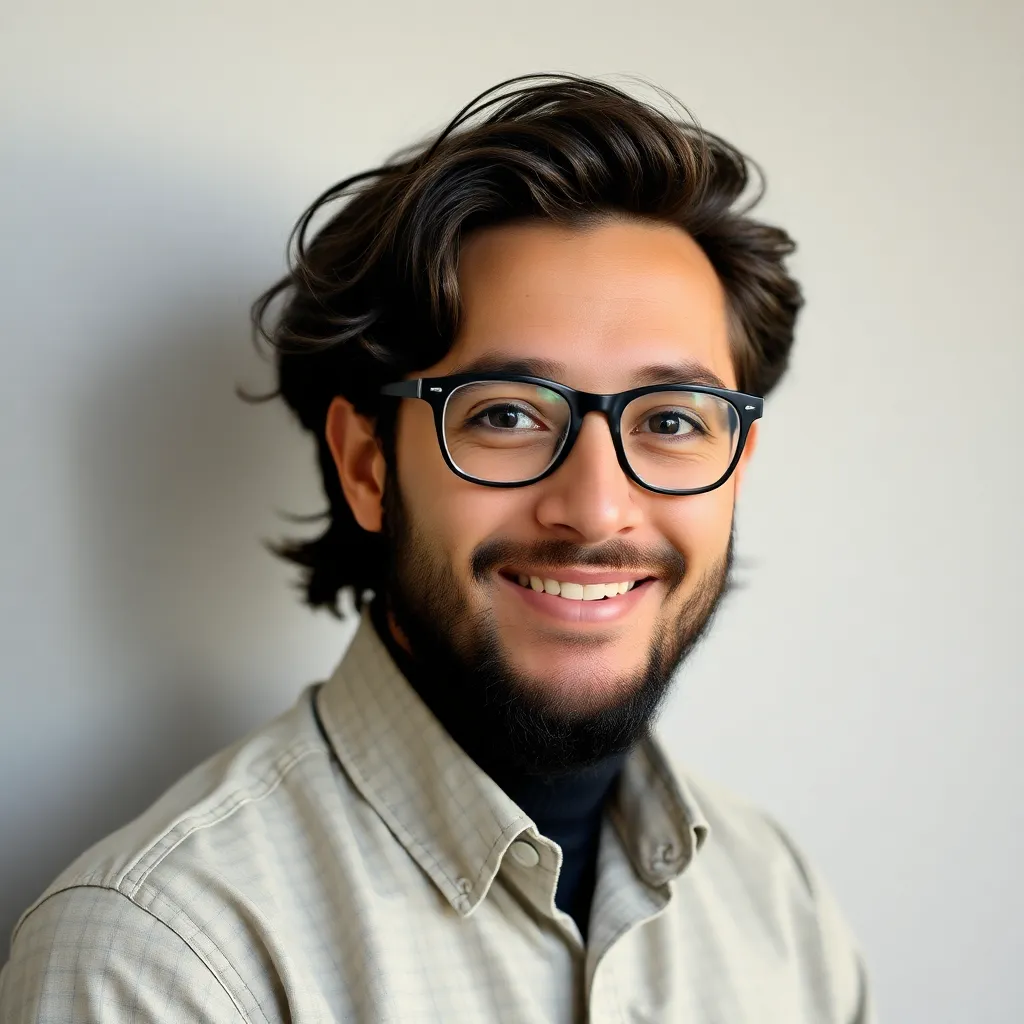
listenit
Mar 12, 2025 · 5 min read

Table of Contents
Do Smaller Molecules Have More Microstates? A Deep Dive into Statistical Thermodynamics
The question of whether smaller molecules possess more microstates than larger ones is not a simple yes or no. The relationship between molecular size and the number of microstates is complex and depends on several factors, primarily the number of atoms, the types of atoms, and the available energy. While intuition might suggest smaller molecules have fewer possibilities, a deeper understanding of statistical thermodynamics reveals a more nuanced picture. This article will explore this relationship, delving into the concepts of microstates, entropy, and the factors influencing the number of accessible microstates for a molecule.
Understanding Microstates and Macrostates
Before diving into the complexities of molecular size and microstates, it’s crucial to define these terms within the context of statistical thermodynamics.
A macrostate describes the overall observable properties of a system, such as temperature, pressure, and volume. For example, a macrostate might describe a gas at a specific temperature and pressure. Importantly, a single macrostate can correspond to a vast number of different microscopic arrangements.
A microstate represents a specific arrangement of the constituent particles (atoms and molecules) of a system. This includes details like the precise positions and momenta of each atom. For even a small system, the number of possible microstates is astronomically large. The macrostate is the average behavior resulting from the collection of all possible microstates.
The Relationship Between Molecular Size and Microstates: The Not-So-Simple Answer
The number of microstates (Ω) available to a system is directly related to its entropy (S) through Boltzmann's equation:
S = k<sub>B</sub>lnΩ
where k<sub>B</sub> is the Boltzmann constant. This means that a system with a higher number of microstates has a higher entropy.
Intuitively, one might assume that smaller molecules have fewer microstates. A diatomic molecule like oxygen (O<sub>2</sub>) has fewer possible configurations than a complex protein with thousands of atoms. However, this is a simplification. The actual number of microstates is influenced by several critical factors:
1. Number of Atoms and Degrees of Freedom:
Larger molecules, with more atoms, possess a greater number of degrees of freedom. Degrees of freedom represent the independent ways a molecule can store energy (translational, rotational, vibrational). Each additional degree of freedom significantly increases the number of accessible energy levels and thus the number of microstates. A simple diatomic molecule has fewer degrees of freedom than a large polyatomic molecule, leading to fewer microstates at the same energy level.
2. Types of Atoms and Bonds:
The types of atoms present and the nature of the bonds between them affect the vibrational modes of the molecule. Different types of atoms have different masses and bond strengths, leading to varying vibrational frequencies and energy levels. This results in a different distribution of microstates. A molecule with a diverse range of atoms and bond types will likely have more microstates than a molecule composed of similar atoms with simpler bonding arrangements.
3. Available Energy:
The available energy plays a decisive role. At low temperatures, only low-energy microstates are accessible. As temperature increases, higher-energy microstates become populated, exponentially increasing the total number of accessible microstates. The increase in accessible microstates with increasing temperature is more pronounced for larger molecules due to their higher number of degrees of freedom.
4. Molecular Flexibility and Conformations:
Large molecules, particularly polymers and biomolecules, often exhibit significant flexibility, leading to numerous possible conformations. Each conformation represents a distinct microstate. This conformational flexibility dramatically increases the number of microstates for larger molecules compared to rigid, smaller molecules. For example, a protein molecule can fold in a vast number of different ways, each representing a distinct microstate.
Illustrative Examples: Comparing Microstates
Let's consider a few examples to illustrate the complexities.
Example 1: Argon (Ar) vs. Carbon Dioxide (CO<sub>2</sub>):
Argon, a monatomic gas, has only translational degrees of freedom. Carbon dioxide, a linear triatomic molecule, has translational, rotational, and vibrational degrees of freedom. At the same temperature and pressure, CO<sub>2</sub> will have significantly more microstates than Ar due to its additional degrees of freedom. However, if we compare them at very low temperatures where only the lowest vibrational levels are accessible, the difference in microstates will be reduced.
Example 2: Methane (CH<sub>4</sub>) vs. a Protein:
Methane, a small molecule, has a relatively small number of microstates compared to a large protein. The protein, with its vast number of atoms and the flexibility resulting from its complex structure, can adopt an astronomical number of conformations. Each of these conformations represents a distinct microstate, leading to a drastically higher number of microstates for the protein.
Implications and Further Considerations
The number of microstates is not solely determined by the number of atoms. It’s a function of energy, temperature, and the intrinsic properties of the molecule, including its structure, bonding, and flexibility. While larger molecules generally possess more degrees of freedom, leading to a higher number of microstates at a given energy, this is not always straightforward. The complexity of the molecule's structure and interactions play a crucial role.
Understanding the relationship between molecular size and microstates has profound implications in various fields:
- Statistical Mechanics: Accurate predictions of thermodynamic properties rely on understanding the number of microstates available to a system.
- Chemical Kinetics: Reaction rates are influenced by the number of microstates involved in the transition state.
- Biochemistry: The vast number of microstates available to biomolecules like proteins contributes to their ability to perform complex functions.
- Materials Science: The properties of materials are intimately connected to the microscopic arrangements of their constituent atoms and molecules.
Conclusion: A More Nuanced Perspective
The simple question of whether smaller molecules have more microstates is not easily answered. While a smaller molecule with fewer atoms and simpler structure might seem to have fewer possibilities, the number of microstates is far more nuanced. It's a function of many factors including the number of atoms and degrees of freedom, the types of atoms and bonds present, the available energy, and the molecular flexibility. Larger molecules, with their greater complexity and degrees of freedom, generally possess a significantly larger number of microstates, particularly at higher temperatures. This complexity underscores the importance of statistical thermodynamics in accurately understanding and predicting the behavior of molecules and systems. The relationship between molecular size and the number of microstates is not simply proportional, but rather a complex interplay of several interacting factors, highlighting the rich and intricate nature of statistical thermodynamics.
Latest Posts
Latest Posts
-
Highest Common Factor Of 8 And 16
May 09, 2025
-
Calculate The Concentration Of Each Solution In Mass Percent
May 09, 2025
-
How Many Atoms Are In Calcium
May 09, 2025
-
9 Minus The Quotient Of 2 And X
May 09, 2025
-
Write 3 3 4 As A Decimal
May 09, 2025
Related Post
Thank you for visiting our website which covers about Do Smaller Molecules Have More Microstates . We hope the information provided has been useful to you. Feel free to contact us if you have any questions or need further assistance. See you next time and don't miss to bookmark.