Distribution Of Relaxation Times Study Of Zinc Oxide
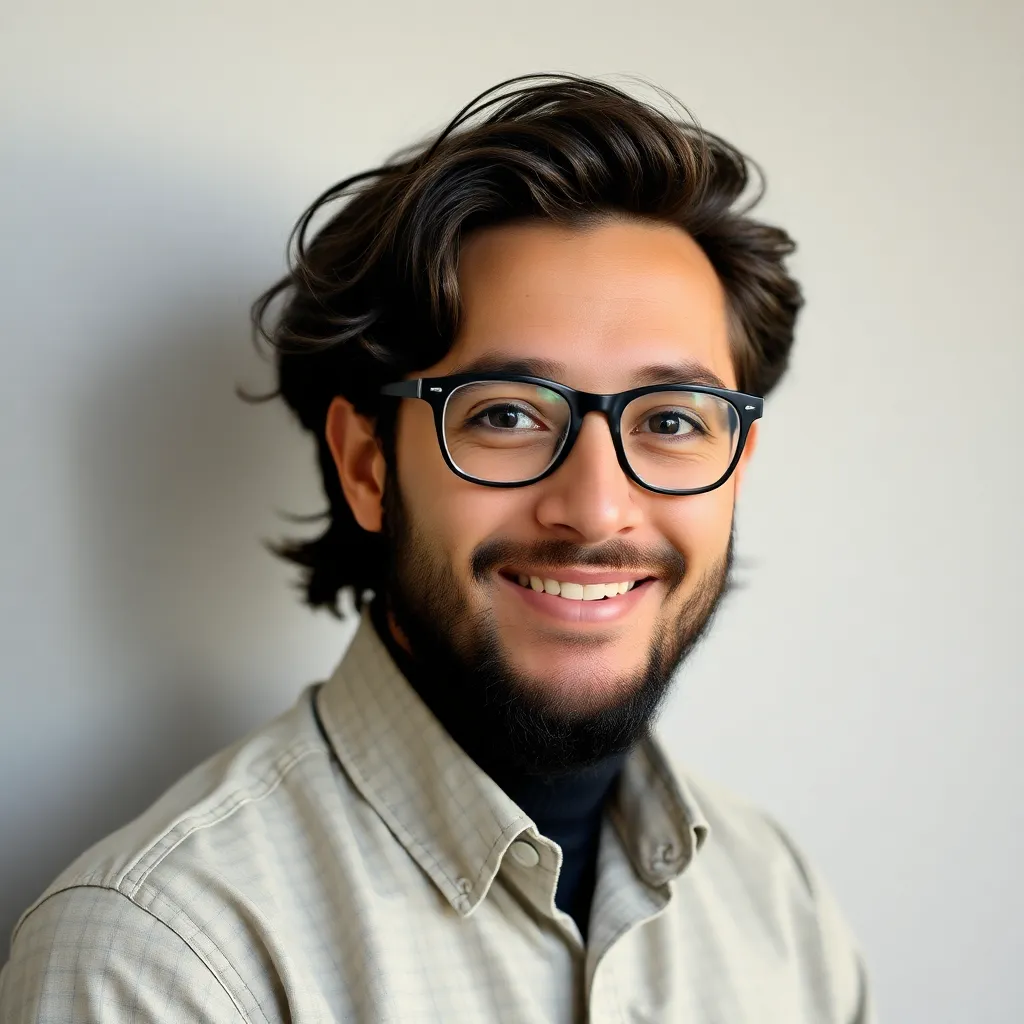
listenit
May 27, 2025 · 6 min read
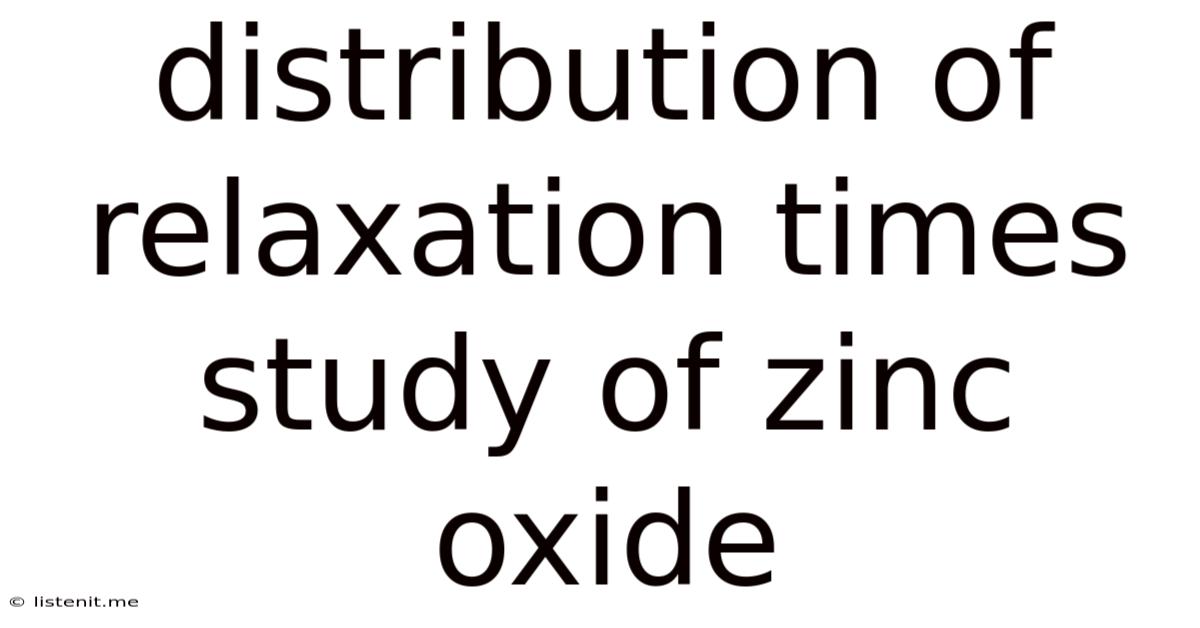
Table of Contents
Distribution of Relaxation Times Study of Zinc Oxide
Zinc oxide (ZnO), a wide-bandgap semiconductor, has garnered significant attention due to its diverse applications in various fields, including electronics, optoelectronics, sensors, and biomedical engineering. Understanding the dielectric properties of ZnO is crucial for optimizing its performance in these applications. A key aspect of dielectric characterization involves the study of the distribution of relaxation times (DRT). This article delves into the intricacies of DRT studies in ZnO, exploring the underlying mechanisms, experimental techniques, and the significance of these studies in advancing our comprehension and application of this versatile material.
What is the Distribution of Relaxation Times (DRT)?
The dielectric response of a material, its ability to store and dissipate electrical energy under an applied electric field, is often not characterized by a single relaxation time. Instead, a distribution of relaxation times is observed, reflecting the heterogeneity of the material's structure and the diverse mechanisms contributing to polarization. This distribution arises from the presence of various types of defects, interfaces, and grain boundaries within the material, each exhibiting distinct relaxation behaviors. The DRT, therefore, provides a detailed picture of the microscopic processes influencing the dielectric response.
Significance of Studying DRT in ZnO
Investigating the DRT in ZnO is paramount for several reasons:
-
Understanding Defect Chemistry: The shape and parameters of the DRT reveal valuable information about the types and concentrations of defects present in the ZnO material. This is crucial for tailoring synthesis methods to control the defect density and ultimately improve the material's properties.
-
Optimizing Device Performance: The dielectric response directly affects the performance of devices based on ZnO, such as capacitors, sensors, and transistors. Understanding the DRT allows for optimization of the material's dielectric properties, thereby enhancing device performance.
-
Investigating Conduction Mechanisms: The DRT study can help elucidate the various conduction mechanisms occurring within ZnO, distinguishing between grain boundary conduction, bulk conduction, and interfacial polarization.
-
Monitoring Material Quality: The DRT acts as a sensitive probe of material quality. Changes in the DRT can indicate the presence of impurities, structural defects, or other imperfections introduced during material processing.
Techniques for Determining the Distribution of Relaxation Times
Several experimental techniques are employed to determine the DRT in ZnO. The most common are:
1. Broadband Dielectric Spectroscopy (BDS)
BDS is a powerful technique that covers a wide range of frequencies (typically from mHz to GHz), allowing for the observation of different relaxation processes. The complex dielectric permittivity (ε* = ε' - jε") is measured as a function of frequency and temperature. From these measurements, the DRT can be extracted using various analytical methods such as:
-
Havriliak-Negami (HN) Function: A widely used empirical function that describes the DRT with several parameters characterizing the relaxation strength, the central relaxation time, and the shape of the distribution.
-
Cole-Cole Function: Another empirical function, simpler than the HN function, suitable for representing relatively narrow DRTs.
-
Inverse Laplace Transform: This mathematical technique allows for the direct determination of the DRT from the measured dielectric data. However, it is often computationally intensive and prone to numerical instabilities.
2. Impedance Spectroscopy (IS)
Similar to BDS, IS measures the impedance (Z*) of the material as a function of frequency and temperature. The impedance data can be analyzed using equivalent circuit models to extract the DRT. This approach is particularly useful for identifying contributions from various elements within the material, such as grains, grain boundaries, and interfaces.
3. Modulus Formalism
Modulus spectroscopy is a complementary approach that emphasizes the relaxation processes in terms of the mechanical response rather than the dielectric response. This offers an alternative perspective on the DRT and can be particularly useful in identifying the contributions from different types of relaxation mechanisms.
Factors Influencing the Distribution of Relaxation Times in ZnO
The DRT in ZnO is significantly influenced by several factors:
1. Grain Size and Grain Boundaries
The presence of grain boundaries significantly affects the dielectric response. Grain boundaries act as barriers to charge transport, leading to a broadening of the DRT. Smaller grain sizes typically result in wider DRTs due to the increased influence of grain boundaries.
2. Defects and Impurities
Point defects such as vacancies, interstitials, and substitutional impurities can introduce localized states within the bandgap, leading to a distribution of relaxation times. The type and concentration of these defects heavily influence the shape and parameters of the DRT.
3. Synthesis Method and Processing Conditions
The synthesis method and processing conditions employed during ZnO preparation significantly influence its microstructure and defect density. Different synthesis methods (e.g., sol-gel, hydrothermal, sputtering) result in variations in the DRT.
4. Temperature
Temperature affects the mobility of charge carriers and the activation energy of different relaxation processes. Therefore, the DRT is highly temperature-dependent, often exhibiting shifts in the central relaxation time and changes in the width of the distribution with varying temperature.
5. Doping
Doping ZnO with various elements can alter the defect chemistry and consequently affect the DRT. Different dopants can introduce different types of defects, leading to variations in the relaxation processes.
Interpretation and Analysis of DRT Data
Analysis of DRT data involves fitting the experimental data to suitable theoretical models, such as the HN or Cole-Cole functions. The fitting parameters provide valuable information regarding the relaxation processes.
-
Relaxation Time (τ): This parameter represents the characteristic time scale of the relaxation process. A shorter relaxation time indicates a faster relaxation process.
-
Distribution Width (α and β): These parameters quantify the width of the DRT. Wider distributions suggest a greater heterogeneity in the relaxation processes.
-
Relaxation Strength: This parameter indicates the magnitude of the contribution of the particular relaxation process to the overall dielectric response.
By carefully analyzing these parameters, one can gain a detailed understanding of the underlying mechanisms responsible for the dielectric behavior of ZnO.
Applications and Future Directions
The understanding of DRT in ZnO has significant implications for various applications:
-
High-k Dielectrics: ZnO's dielectric properties make it a promising candidate for high-k dielectrics in advanced electronic devices. Understanding the DRT is essential for optimizing its performance in these applications.
-
Gas Sensors: ZnO-based gas sensors rely on changes in their dielectric properties upon exposure to different gases. Understanding the DRT can help improve the sensitivity and selectivity of these sensors.
-
Varistors: ZnO varistors, used for voltage surge protection, exhibit a nonlinear current-voltage characteristic dependent on their dielectric properties. Studies of DRT are crucial in optimizing their performance.
-
Photocatalysis: The dielectric properties of ZnO play a role in its photocatalytic activity. Understanding the DRT can aid in optimizing its efficiency in various photocatalytic applications.
Future research directions in the DRT study of ZnO include:
-
Advanced Modeling Techniques: Developing more sophisticated theoretical models to accurately capture the complexity of the relaxation processes in ZnO.
-
Correlation with Microstructure: Establishing a strong correlation between the DRT and the microstructure of ZnO using advanced characterization techniques.
-
Impact of Surface Modifications: Investigating the effect of surface modifications on the DRT and how this can be utilized for tailoring the properties of ZnO.
-
Exploration of Novel ZnO-based Composites: Studying the DRT of novel ZnO-based composites to explore new applications.
In conclusion, the distribution of relaxation times study of ZnO is a crucial aspect of understanding its dielectric properties and optimizing its performance in diverse applications. By employing various experimental techniques and employing appropriate theoretical models, researchers can gain valuable insights into the microscopic processes governing the dielectric response of ZnO, paving the way for its further development and wider applications in advanced materials and technologies. Continued research in this field is essential to unlocking the full potential of this versatile material.
Latest Posts
Latest Posts
-
What Is The Function Of The Setae
May 28, 2025
-
Which Of The Following Techniques Is Used In Auto Transfusion
May 28, 2025
-
The Factors Associated With Creativity Include
May 28, 2025
-
The Sexual Rights Of Children M Cook Constantine
May 28, 2025
-
First Laboratory Of Psychology Was Established By
May 28, 2025
Related Post
Thank you for visiting our website which covers about Distribution Of Relaxation Times Study Of Zinc Oxide . We hope the information provided has been useful to you. Feel free to contact us if you have any questions or need further assistance. See you next time and don't miss to bookmark.