Describe The Three Parts Of An Atp Molecule
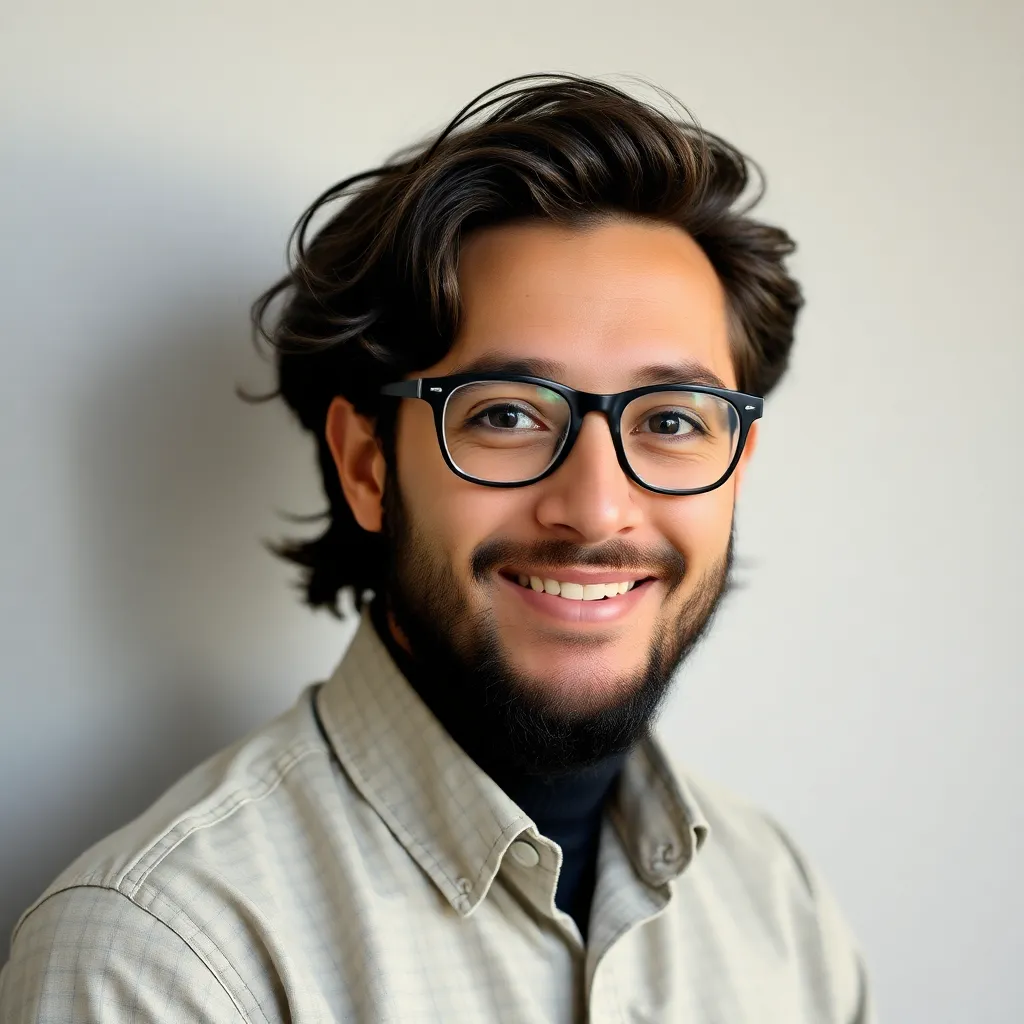
listenit
May 12, 2025 · 6 min read
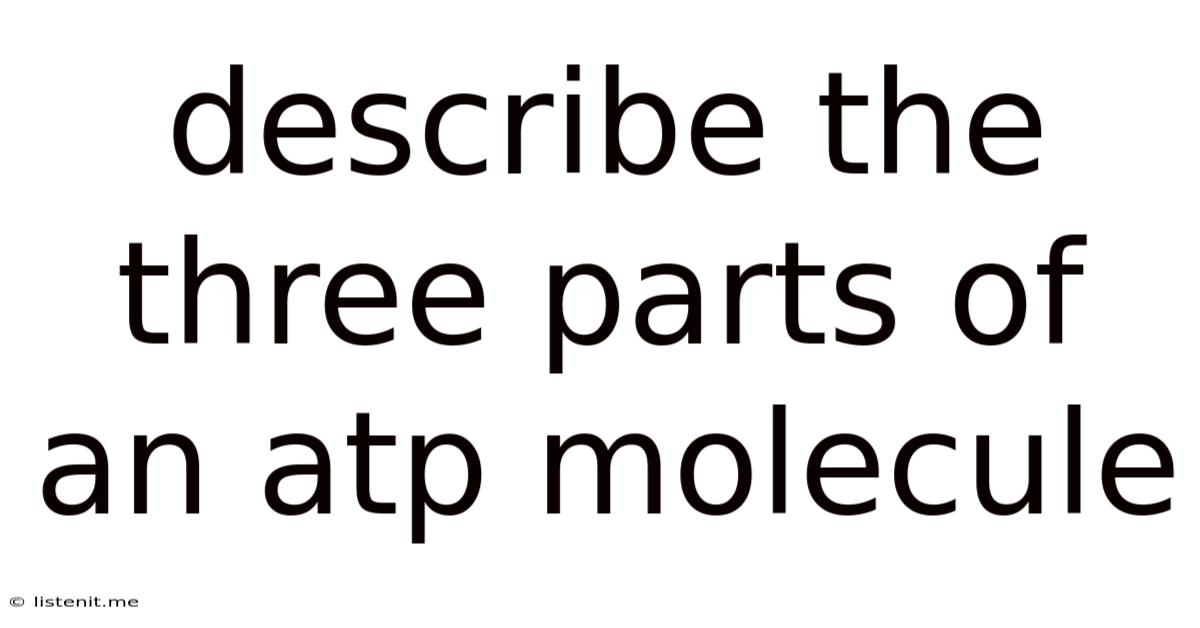
Table of Contents
Delving Deep into ATP: A Comprehensive Look at its Three-Part Structure
Adenosine triphosphate (ATP) is the powerhouse of the cell, the primary energy currency that fuels countless biological processes. Understanding its structure is crucial to grasping its function. This article will provide a comprehensive overview of ATP's three components: adenine, ribose, and the triphosphate group, exploring their individual properties and how they collectively contribute to ATP's remarkable energy-carrying capabilities. We'll also touch upon the significance of ATP in various biological contexts and its crucial role in maintaining life.
The Three Pillars of ATP: A Detailed Examination
ATP's structure can be visualized as a three-part molecule, intricately assembled to facilitate energy transfer within the cell. These three parts are:
1. Adenine: The Nitrogenous Base
Adenine is a purine base, a type of nitrogen-containing organic molecule with a double-ring structure. This structure is crucial for its ability to form hydrogen bonds with its complementary base, thymine (in DNA) or uracil (in RNA). However, in ATP, adenine's role extends beyond base pairing. Its aromatic nature contributes to the molecule's overall stability and its ability to participate in various interactions within the cellular environment. The specific arrangement of nitrogen and carbon atoms in adenine's structure directly influences its electron distribution, making it a vital component in the energy transfer processes facilitated by ATP. The planarity of the adenine ring also contributes to the overall structure and stability of the ATP molecule, influencing its interactions with enzymes and other cellular components. The presence of nitrogen atoms provides sites for hydrogen bonding, influencing interactions with other molecules and contributing to the molecule's stability.
Key features of adenine in ATP:
- Purine base: A double-ringed structure providing stability.
- Nitrogenous nature: Allows for hydrogen bonding interactions.
- Planar structure: Contributes to the overall structural integrity of ATP.
- Electron distribution: Plays a role in energy transfer reactions.
2. Ribose: The Pentose Sugar
Ribose is a five-carbon sugar (pentose) that forms the backbone of ATP. Specifically, it's a β-D-ribose, meaning the hydroxyl group (-OH) on the anomeric carbon (carbon 1) is in the beta configuration and points upwards. This specific configuration is crucial for the molecule's overall three-dimensional shape and how it interacts with other molecules. The ribose sugar is linked to the adenine base through a glycosidic bond, creating a nucleoside called adenosine. The ribose sugar's hydroxyl groups can participate in hydrogen bonding, which influences the interactions of ATP with enzymes and other molecules. The ring structure of the ribose adds to the stability of the molecule, while the hydroxyl groups provide sites for chemical modifications and interactions. The precise spatial arrangement of the atoms in the ribose molecule is essential for its function as a component of ATP.
Key features of ribose in ATP:
- Pentose sugar: A five-carbon ring providing structural support.
- β-D-ribose configuration: Specific stereochemistry crucial for functionality.
- Hydroxyl groups: Sites for hydrogen bonding and potential modifications.
- Glycosidic linkage: Connects ribose to adenine, forming adenosine.
3. Triphosphate Group: The Energy Reservoir
The triphosphate group (α, β, and γ phosphates) is arguably the most critical component of ATP, representing the molecule's primary energy storage site. The three phosphate groups are linked together by high-energy phosphoanhydride bonds. These bonds are characterized by a relatively high energy of hydrolysis, meaning that the energy released upon breaking these bonds is significantly higher than that released from other phosphate bonds. This high energy release is what fuels numerous cellular processes. The negatively charged phosphate groups repel each other due to electrostatic repulsion, creating inherent instability within the triphosphate chain. This inherent instability is crucial; the energy stored in ATP is, in essence, the potential energy of these repelling phosphate groups. Hydrolysis of the phosphoanhydride bonds—typically the terminal phosphoanhydride bond between the β and γ phosphates—releases this stored energy, driving cellular work. The release of energy is coupled to various enzymatic reactions, making ATP a vital intermediary in cellular energy transduction.
Key features of the triphosphate group in ATP:
- Three phosphate groups: Linked by high-energy phosphoanhydride bonds.
- High-energy bonds: Hydrolysis releases significant amounts of energy.
- Electrostatic repulsion: Contributes to the instability and stored energy.
- Energy coupling: Enables ATP to drive various cellular processes.
ATP's Role in Cellular Processes: A Multifaceted Powerhouse
ATP's versatility extends across a vast range of cellular functions. Its hydrolysis powers a multitude of processes, including:
- Muscle contraction: ATP provides the energy for the interaction between actin and myosin filaments, enabling muscle movement.
- Active transport: ATP drives the movement of molecules against their concentration gradients across cell membranes, essential for maintaining cellular homeostasis.
- Biosynthesis: ATP provides the energy needed for the synthesis of complex molecules, including proteins, nucleic acids, and lipids.
- Nerve impulse transmission: ATP is involved in the generation and propagation of nerve impulses, crucial for communication within the nervous system.
- Signal transduction: ATP acts as a signaling molecule in several cellular pathways, regulating various cellular processes.
- DNA replication and repair: ATP is a crucial component in the processes of DNA replication and repair, maintaining the integrity of the genome.
- Protein folding and degradation: ATP fuels chaperone proteins that assist in proper protein folding and the degradation of misfolded or damaged proteins.
- Cellular motility: ATP powers the movement of cilia and flagella, enabling cell locomotion and movement of substances across cell surfaces.
The constant cycling between ATP and ADP (adenosine diphosphate) is essential for maintaining the cellular energy balance. ATP is continuously generated through cellular respiration (oxidative phosphorylation) and other metabolic pathways, while its hydrolysis releases energy to fuel cellular work. The efficiency of this cycle is crucial for optimal cellular function. Disruptions in ATP production or utilization can lead to various cellular dysfunctions and diseases.
The Significance of ATP Hydrolysis and Phosphorylation
The reversible conversion between ATP and ADP (adenosine diphosphate) is at the heart of cellular energy metabolism. ATP hydrolysis, the breaking of a phosphoanhydride bond, releases energy, typically around -7.3 kcal/mol, a value that can vary slightly depending on conditions. This energy is then utilized to drive endergonic (energy-requiring) reactions. Phosphorylation, on the other hand, involves the transfer of a phosphate group from ATP to another molecule, often a protein. This process can alter the conformation of the protein, activating it or changing its function, often making an energetically unfavorable reaction possible. These processes are intricately linked and highly regulated, ensuring that energy is used efficiently and appropriately within the cell.
The energy released during ATP hydrolysis is not directly used to power cellular work. Instead, it's coupled to other reactions through enzyme catalysis. Enzymes often use the released phosphate group to change the shape of the target molecule, activating it and enabling the reaction to occur. This coupling of ATP hydrolysis to other reactions is critical for the efficient energy utilization within the cell, ensuring that energy is transferred in a controlled and precise manner.
Conclusion: ATP – The Cell's Indispensable Energy Currency
Adenosine triphosphate, with its intricate three-part structure, stands as the quintessential energy molecule of life. The adenine base, ribose sugar, and triphosphate group work in concert, creating a molecule capable of efficiently storing and releasing energy to fuel the countless processes that sustain life. Understanding the details of its structure is fundamental to comprehending the intricacies of cellular metabolism and the remarkable efficiency of energy transduction within living organisms. Future research into ATP and its associated processes promises to yield further insights into cellular function and the development of therapeutic strategies for various diseases linked to impaired energy metabolism. The ongoing study of ATP underscores its central role in biology and its continued importance as a key area of investigation in the life sciences.
Latest Posts
Latest Posts
-
What Is A Compound Predicate Sentence
May 12, 2025
-
Calculate The Value Of The Rate Constant K
May 12, 2025
-
A Homeotic Gene Is One That Might Affect
May 12, 2025
-
How To Calculate The Mass Of A Cube
May 12, 2025
-
What Is The Lcm Of 30 And 40
May 12, 2025
Related Post
Thank you for visiting our website which covers about Describe The Three Parts Of An Atp Molecule . We hope the information provided has been useful to you. Feel free to contact us if you have any questions or need further assistance. See you next time and don't miss to bookmark.