Cellular Respiration Uses 1 Molecule Of Glucose To Produce Approximately
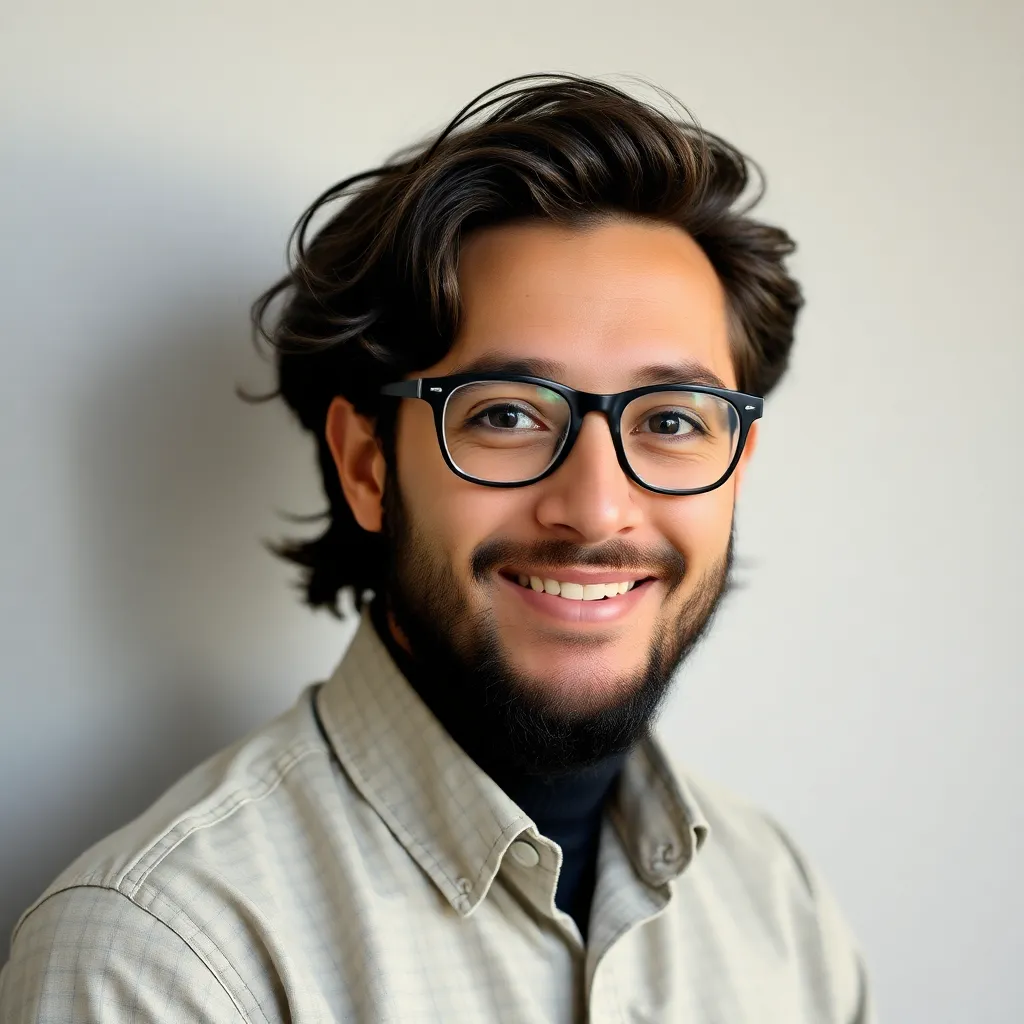
listenit
May 09, 2025 · 6 min read
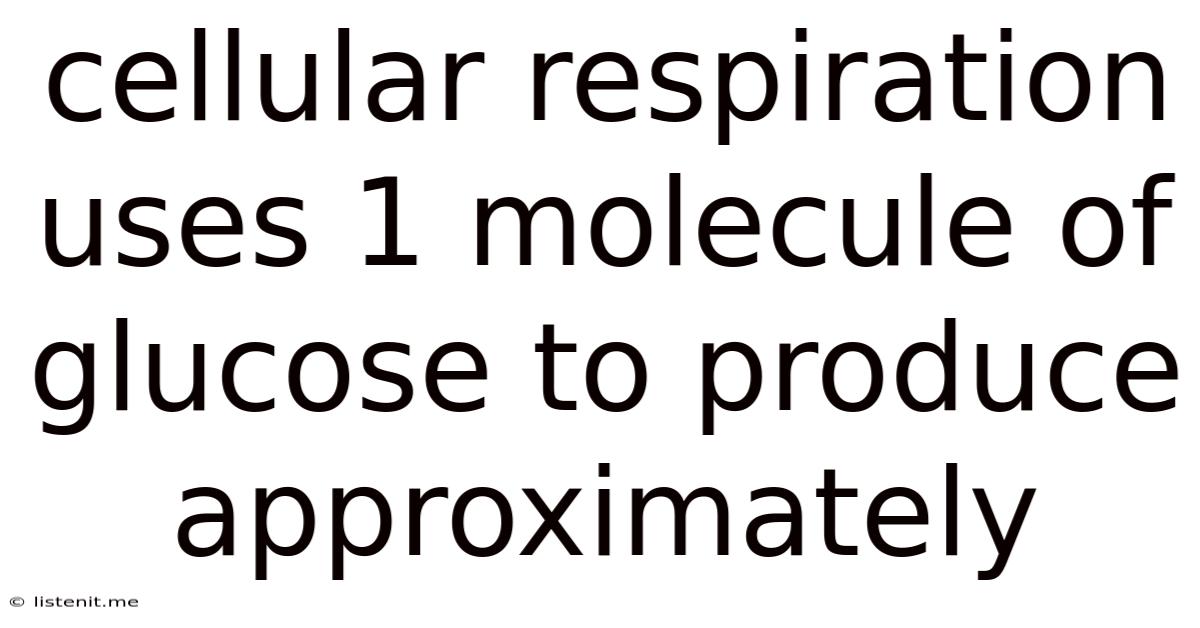
Table of Contents
Cellular Respiration: The Glucose-to-ATP Conversion and Its Efficiency
Cellular respiration is the fundamental process by which living organisms convert the chemical energy stored in glucose into a readily usable form of energy called adenosine triphosphate (ATP). This intricate process, occurring within the mitochondria of eukaryotic cells, is crucial for powering all cellular activities, from muscle contraction to protein synthesis. While often simplified as a single equation, the reality is far more complex and nuanced. This article will delve into the details of cellular respiration, examining how one molecule of glucose yields approximately 30-32 ATP molecules, exploring the various stages involved, and discussing factors influencing the final ATP yield.
The Big Picture: Glucose to ATP
The overall equation for cellular respiration is commonly represented as:
C₆H₁₂O₆ + 6O₂ → 6CO₂ + 6H₂O + ATP
This equation concisely summarizes the process: glucose (C₆H₁₂O₆) reacts with oxygen (O₂) to produce carbon dioxide (CO₂), water (H₂O), and, importantly, ATP. However, this simplification masks the multi-step pathway and the intricate mechanisms involved in ATP generation. The actual number of ATP molecules produced from one glucose molecule isn't a fixed 30-32 but rather a range. This variation stems from several factors, which we’ll explore later.
The Stages of Cellular Respiration: A Detailed Breakdown
Cellular respiration is broadly divided into four main stages:
1. Glycolysis: The First Steps in Glucose Breakdown
Glycolysis, meaning "splitting of sugar," occurs in the cytoplasm and doesn't require oxygen (anaerobic). It's the initial breakdown of glucose into two molecules of pyruvate (a three-carbon compound). This stage yields a net gain of 2 ATP molecules and 2 NADH molecules (electron carriers). Crucially, this stage sets the stage for subsequent, more energy-yielding processes.
2. Pyruvate Oxidation: Preparing for the Krebs Cycle
Pyruvate, the product of glycolysis, is transported into the mitochondria. Here, it undergoes oxidative decarboxylation, a reaction that removes a carbon atom as carbon dioxide (CO₂) and converts pyruvate into acetyl-CoA. This step also generates one NADH molecule per pyruvate, resulting in a total of 2 NADH molecules for one glucose molecule (since two pyruvates are formed from one glucose).
3. The Krebs Cycle (Citric Acid Cycle): Central Hub of Energy Production
The acetyl-CoA generated in pyruvate oxidation enters the Krebs cycle, a cyclical series of reactions within the mitochondrial matrix. For each acetyl-CoA molecule (two per glucose molecule), the Krebs cycle produces:
- 1 ATP molecule (through substrate-level phosphorylation).
- 3 NADH molecules.
- 1 FADH₂ molecule (another electron carrier).
Since two acetyl-CoA molecules are produced from one glucose, the total yield from the Krebs cycle for one glucose is 2 ATP, 6 NADH, and 2 FADH₂.
4. Oxidative Phosphorylation: The Electron Transport Chain and Chemiosmosis
This stage, occurring in the inner mitochondrial membrane, is where the majority of ATP is generated. The NADH and FADH₂ molecules generated in the previous stages donate their electrons to the electron transport chain (ETC), a series of protein complexes embedded in the inner mitochondrial membrane.
As electrons move down the ETC, energy is released, used to pump protons (H⁺) from the mitochondrial matrix to the intermembrane space, creating a proton gradient. This gradient represents potential energy. The protons then flow back into the matrix through ATP synthase, an enzyme that uses this proton motive force to synthesize ATP. This process is called chemiosmosis.
The ATP Yield: A More Accurate Calculation
The commonly cited figure of 30-32 ATP molecules per glucose molecule is an approximation. The actual yield varies depending on several factors:
-
Shuttle systems: NADH generated during glycolysis needs to be transported into the mitochondria. Different shuttle systems exist (e.g., glycerol-3-phosphate shuttle, malate-aspartate shuttle), and their efficiency varies, affecting the number of ATP molecules produced from each NADH. The malate-aspartate shuttle is more efficient, yielding more ATP.
-
Proton leak: Some protons can leak across the inner mitochondrial membrane, bypassing ATP synthase and reducing the ATP yield.
-
ATP consumption: Some ATP is used in the transport of molecules into and out of the mitochondria, further reducing the net ATP gain.
Considering these factors, a more nuanced calculation could yield:
- Glycolysis: 2 ATP + 2 NADH (approximately 5 ATP if using the malate-aspartate shuttle)
- Pyruvate oxidation: 2 NADH (approximately 5 ATP)
- Krebs cycle: 2 ATP + 6 NADH (approximately 15 ATP) + 2 FADH₂ (approximately 3 ATP)
Total: Approximately 30-32 ATP
This shows that the majority of ATP production occurs during oxidative phosphorylation.
Factors Affecting Cellular Respiration Efficiency
Several factors can influence the efficiency of cellular respiration and, consequently, the ATP yield:
-
Oxygen availability: Cellular respiration is an aerobic process; the absence of oxygen leads to anaerobic respiration (fermentation), which yields significantly less ATP.
-
Temperature: Enzyme activity is temperature-dependent. Optimal temperatures maximize enzyme function and ATP production, while extreme temperatures can denature enzymes and reduce efficiency.
-
pH: Changes in pH can affect enzyme activity and the integrity of mitochondrial membranes, impacting ATP production.
-
Nutrient availability: The availability of glucose and other substrates is essential for cellular respiration. Limited substrate availability will limit ATP production.
-
Genetic factors: Mutations affecting mitochondrial genes or enzymes involved in cellular respiration can reduce ATP production.
Beyond Glucose: Other Energy Sources
While glucose is the primary fuel source for cellular respiration, other molecules can also be utilized. These include:
-
Fatty acids: Beta-oxidation breaks down fatty acids into acetyl-CoA, which enters the Krebs cycle. Fatty acids generally yield significantly more ATP than glucose per carbon atom.
-
Amino acids: Amino acids can be converted into various intermediates of glycolysis and the Krebs cycle, contributing to ATP production.
Cellular Respiration and Human Health
Dysfunction in cellular respiration can have significant health consequences. Mitochondrial diseases, caused by mutations affecting mitochondrial genes or proteins involved in cellular respiration, can lead to a wide range of symptoms affecting multiple organ systems. Moreover, impaired cellular respiration is implicated in various conditions, including:
-
Cancer: Cancer cells often exhibit altered metabolism, including changes in cellular respiration.
-
Neurodegenerative diseases: Mitochondrial dysfunction plays a role in diseases like Alzheimer's and Parkinson's.
-
Diabetes: Impaired glucose metabolism and cellular respiration can contribute to diabetes.
Conclusion
Cellular respiration is a marvel of biological engineering, efficiently converting the chemical energy stored in glucose into the readily usable form of ATP that powers life. While the simplified equation suggests a straightforward process, the reality is a multi-step pathway with intricate regulation and numerous factors influencing the final ATP yield. Understanding this complex process is crucial for comprehending the basis of life and for investigating the roles of mitochondrial dysfunction in various human diseases. The approximate 30-32 ATP molecules generated per glucose molecule represent a remarkable energy harvest, essential for maintaining the dynamism of cellular life. Further research continues to unravel the subtleties of this essential biological process, leading to advancements in our understanding of health and disease.
Latest Posts
Latest Posts
-
Eight Minus The Square Of A Number
May 11, 2025
-
800 Is 1 10 Of What Number
May 11, 2025
-
How To Find The Relative Minimum
May 11, 2025
-
Elements In The Same Group Or Family Share Important
May 11, 2025
-
The Water Cycle Is Powered By The
May 11, 2025
Related Post
Thank you for visiting our website which covers about Cellular Respiration Uses 1 Molecule Of Glucose To Produce Approximately . We hope the information provided has been useful to you. Feel free to contact us if you have any questions or need further assistance. See you next time and don't miss to bookmark.