Ammonia Is Formed From Its Elements
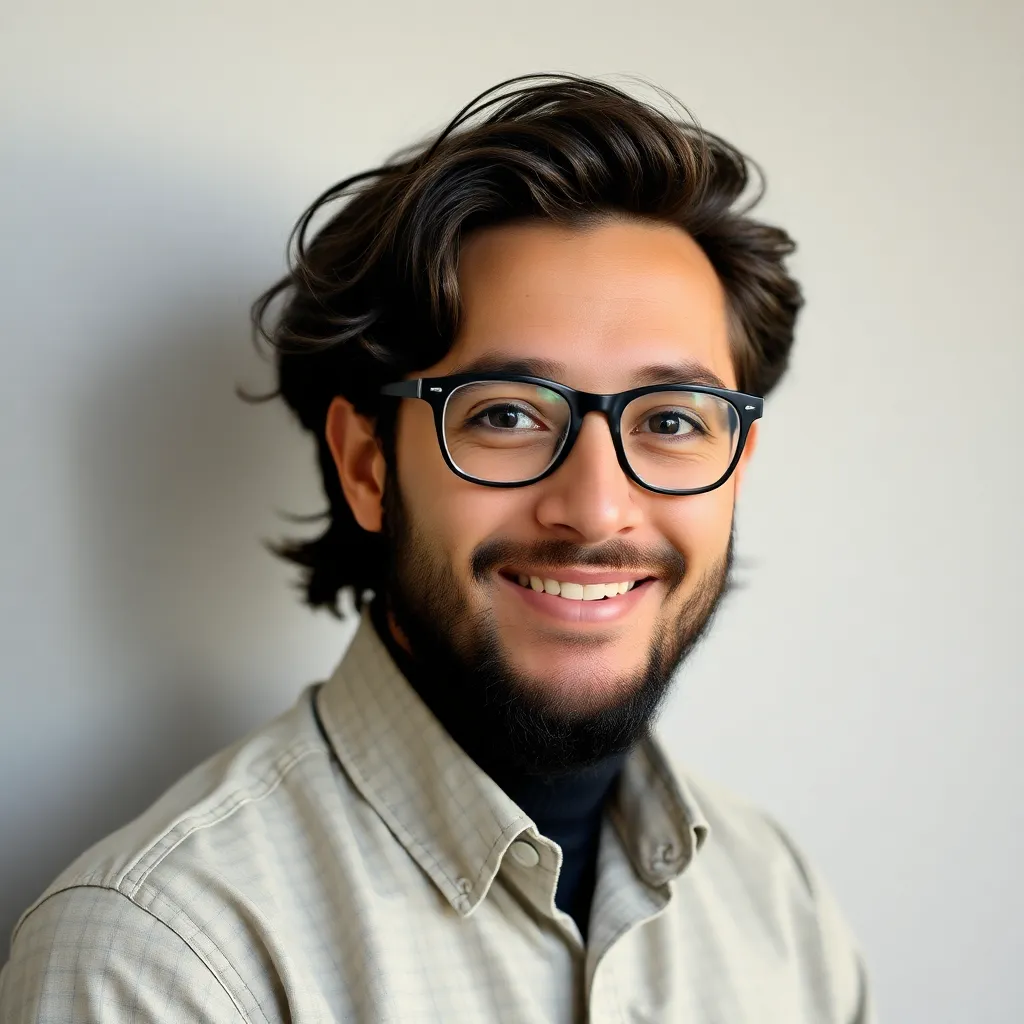
listenit
May 09, 2025 · 5 min read
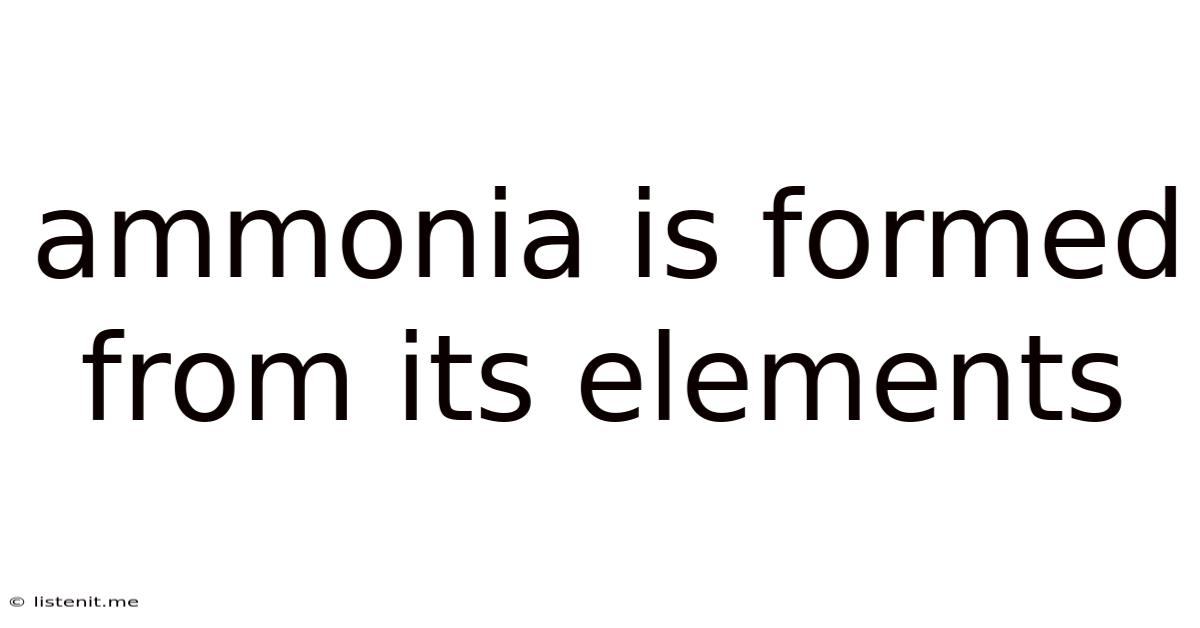
Table of Contents
Ammonia: Formation from its Elements – A Deep Dive into the Haber-Bosch Process and Beyond
Ammonia (NH₃), a colorless gas with a pungent odor, is a cornerstone chemical in various industries, from fertilizers to pharmaceuticals. Understanding its formation from its constituent elements – nitrogen (N₂) and hydrogen (H₂) – is crucial to appreciating its significance and the complex processes involved. This article will delve into the intricacies of ammonia synthesis, focusing primarily on the Haber-Bosch process, its history, challenges, and ongoing efforts toward optimization and sustainability.
The Haber-Bosch Process: A Revolution in Fertilizer Production
The Haber-Bosch process, named after Fritz Haber and Carl Bosch, revolutionized ammonia production in the early 20th century. Before its development, ammonia synthesis was a significant challenge, with limited and inefficient methods available. The Haber-Bosch process enabled the large-scale, industrial production of ammonia, drastically impacting global food security by providing the crucial nitrogen needed for fertilizer production.
The Chemistry Behind the Synthesis: N₂ + 3H₂ ⇌ 2NH₃
The fundamental reaction at the heart of the Haber-Bosch process is the reversible combination of nitrogen and hydrogen gases to form ammonia:
N₂(g) + 3H₂(g) ⇌ 2NH₃(g)
This seemingly simple equation hides a complex reality. The reaction is:
- Exothermic: The forward reaction (formation of ammonia) releases heat.
- Equilibrium-Limited: The reaction reaches an equilibrium state, meaning that at a given temperature and pressure, the rate of ammonia formation equals the rate of ammonia decomposition.
- Challenging Kinetics: The reaction is kinetically slow, meaning that it requires a catalyst to proceed at a reasonable rate.
Understanding the Reaction Conditions: A Balancing Act
To maximize ammonia yield, the Haber-Bosch process carefully balances several critical reaction parameters:
-
Temperature: Lower temperatures favor the forward reaction (higher ammonia yield) as it's exothermic. However, lower temperatures also slow down the reaction rate. A compromise temperature of around 450-500°C is typically used.
-
Pressure: High pressure favors the forward reaction because it reduces the number of gas molecules (4 on the reactant side, 2 on the product side). Pressures ranging from 150 to 350 atmospheres are commonly employed.
-
Catalyst: An iron-based catalyst is crucial to accelerate the reaction rate. Promoters, such as aluminum oxide (Al₂O₃) and potassium oxide (K₂O), are added to enhance the catalyst's efficiency. The catalyst provides an alternative reaction pathway with lower activation energy.
-
Gas Purification: High purity nitrogen and hydrogen feedstock are essential to prevent catalyst poisoning and maintain efficient operation. Impurities can significantly reduce catalyst activity and overall ammonia yield.
The Importance of the Haber-Bosch Process: Feeding the World
The impact of the Haber-Bosch process on global food production cannot be overstated. Before its invention, nitrogen fertilizers were scarce and expensive. This limited agricultural yields, hindering the ability to feed a rapidly growing global population. The Haber-Bosch process made nitrogen fertilizers readily available and affordable, leading to a dramatic increase in crop yields and contributing significantly to the "green revolution." It's estimated that approximately 50% of the nitrogen in our bodies originates from ammonia produced via this process.
Challenges and Future Directions: Sustainability and Optimization
Despite its revolutionary impact, the Haber-Bosch process faces several challenges:
-
Energy Intensive: The process is energy-intensive, consuming a significant amount of fossil fuels, mainly natural gas, to generate the high temperatures and pressures required. This contributes significantly to greenhouse gas emissions.
-
Environmental Concerns: The production of hydrogen, often from natural gas via steam reforming, is a significant source of carbon dioxide (CO₂) emissions. Ammonia production is thus intricately linked to climate change.
-
Catalyst Deactivation: The iron-based catalyst gradually deactivates over time, requiring periodic replacement. Research focuses on developing more robust and longer-lasting catalysts.
Strides Towards a Greener Ammonia Synthesis:
Researchers are actively exploring alternative methods for ammonia synthesis that address the environmental concerns associated with the Haber-Bosch process:
-
Electrochemical Synthesis: Electrochemical methods use renewable energy sources (solar, wind, hydro) to power the synthesis of ammonia directly from nitrogen and water, eliminating the need for fossil fuels. This approach, while promising, faces challenges in efficiency and scalability.
-
Photocatalytic Synthesis: Photocatalysis uses sunlight to drive the ammonia synthesis reaction, offering a pathway towards a truly sustainable process. Developing efficient and stable photocatalysts is a key research area.
-
Bio-Inspired Ammonia Synthesis: Nature provides inspiration through nitrogen-fixing bacteria that can convert atmospheric nitrogen into ammonia at ambient temperature and pressure. Understanding the intricate mechanisms of these bacteria could lead to bio-inspired catalytic systems for ammonia synthesis.
-
Improved Catalyst Design: Ongoing research focuses on improving the efficiency and lifespan of catalysts. This includes exploring novel materials and optimizing catalyst structure and composition.
-
Process Intensification: Process intensification aims to reduce energy consumption and improve efficiency by optimizing reaction conditions and employing more compact reactor designs.
Beyond Fertilizers: Ammonia's Expanding Role
Ammonia's applications extend far beyond fertilizer production. It is a vital building block for numerous industrial chemicals, including:
- Nitric Acid (HNO₃): A key component in the production of fertilizers, explosives, and other chemicals.
- Urea [(NH₂)₂CO]: A widely used nitrogenous fertilizer.
- Hydrazine (N₂H₄): Used as a rocket propellant and in various industrial applications.
- Polymers and Plastics: Ammonia serves as a precursor for certain polymers and plastics.
- Pharmaceuticals: Ammonia is used in the synthesis of various pharmaceuticals.
Conclusion: A Continuous Quest for Optimization
The Haber-Bosch process stands as a testament to human ingenuity and its profound impact on global food security. However, its environmental footprint necessitates a continuous search for more sustainable and efficient methods of ammonia synthesis. Ongoing research efforts, focusing on electrochemical, photocatalytic, and bio-inspired approaches, hold great promise in delivering a greener and more sustainable future for ammonia production, ensuring its continued crucial role in meeting the world's growing demands. The quest for a greener ammonia synthesis is not just about improving efficiency, but about ensuring a sustainable future for generations to come. The fundamental chemistry remains the same, but the methods of achieving it are constantly evolving to meet the challenges of the 21st century.
Latest Posts
Latest Posts
-
How Many Valence Electrons Do Carbon Have
May 09, 2025
-
What Is 3 Over 5 As A Decimal
May 09, 2025
-
Naoh And Potassium Hydrogen Phthalate Reaction
May 09, 2025
-
Elements That Have The Same Number Of Valence Electrons Are
May 09, 2025
-
Which Innovation Allowed For The Mass Production Of Goods
May 09, 2025
Related Post
Thank you for visiting our website which covers about Ammonia Is Formed From Its Elements . We hope the information provided has been useful to you. Feel free to contact us if you have any questions or need further assistance. See you next time and don't miss to bookmark.