Absolute Zero Is What Temperature On The Fahrenheit Scale
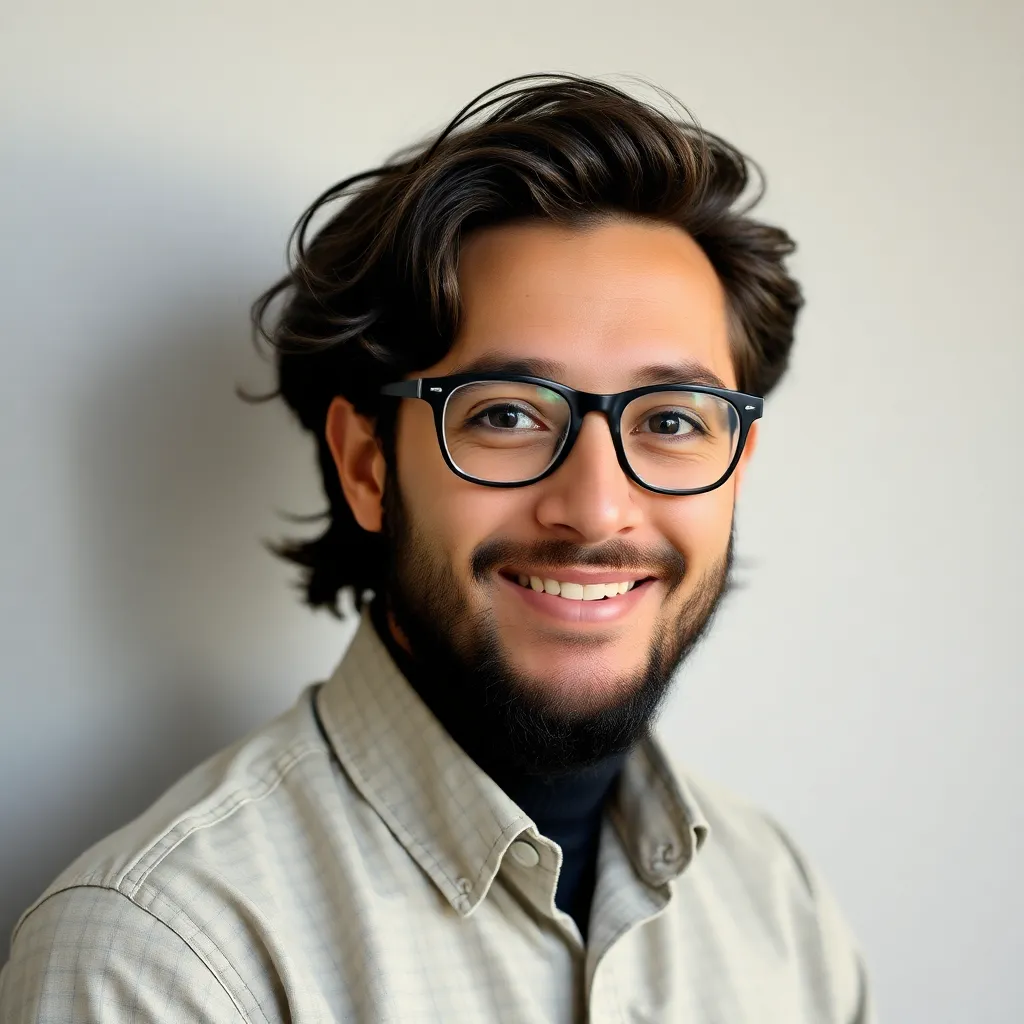
listenit
May 10, 2025 · 5 min read
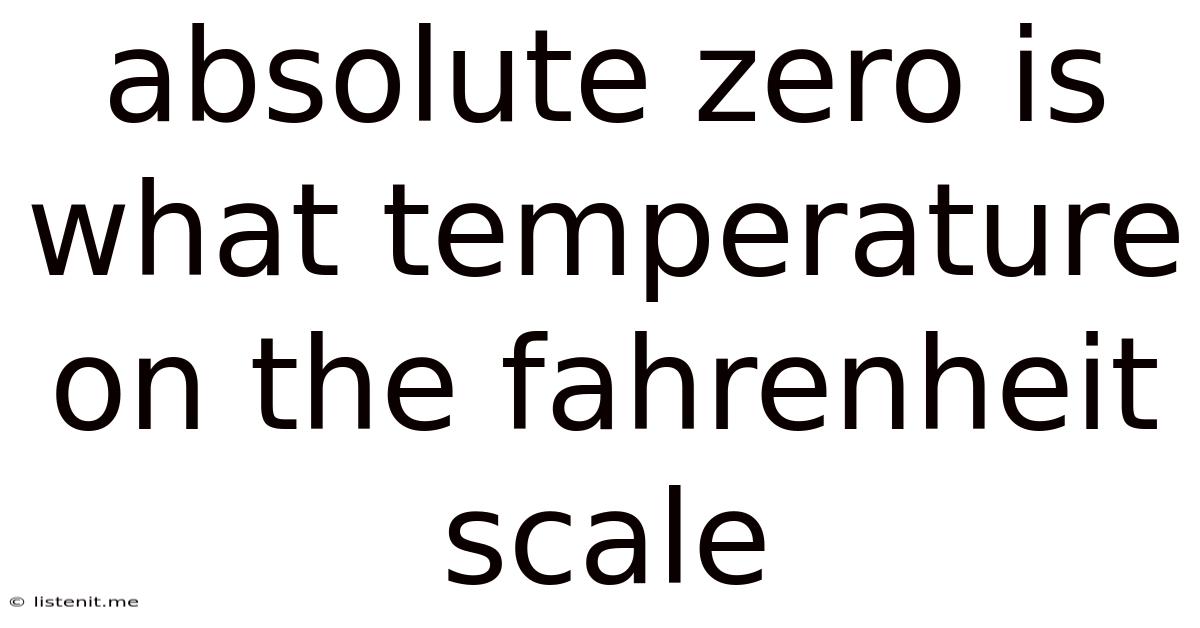
Table of Contents
Absolute Zero: What Temperature is it on the Fahrenheit Scale?
Absolute zero, the theoretical point at which all molecular motion ceases, holds a captivating position in the world of physics and thermodynamics. While conceptually straightforward, understanding its temperature on various scales, especially the Fahrenheit scale, requires a grasp of the different temperature scales and their relationships. This article delves deep into the concept of absolute zero, explains its significance, and meticulously calculates its equivalent on the Fahrenheit scale. We’ll also explore related concepts like the Kelvin scale and the implications of reaching absolute zero.
Understanding Absolute Zero
Absolute zero represents the lowest possible temperature, the point at which a substance possesses no thermal energy. It's not merely the absence of heat; it's a fundamental limit defined by the laws of thermodynamics. At this point, all atoms and molecules would possess minimal vibrational and translational energy, theoretically achieving a state of perfect stillness. This is a theoretical concept, as reaching absolute zero in practice is impossible due to the inherent limitations imposed by quantum mechanics. Even at incredibly low temperatures, minute residual energy persists.
The significance of absolute zero extends far beyond theoretical physics. It serves as the foundation for various scientific models and calculations, particularly those involving thermodynamics and statistical mechanics. Understanding absolute zero helps us comprehend the behavior of materials at extremely low temperatures, leading to advancements in fields like cryogenics and superconducting technologies.
Why is reaching absolute zero impossible?
The Third Law of Thermodynamics states that it is impossible to reach absolute zero in a finite number of steps. This is due to the quantum mechanical nature of energy. Even at near-absolute zero temperatures, particles still possess some residual energy, known as zero-point energy. This energy is a consequence of the Heisenberg Uncertainty Principle, which states that we cannot simultaneously know both the position and momentum of a particle with perfect accuracy. This inherent uncertainty leads to a minimum energy level that cannot be reduced to zero.
Temperature Scales: Celsius, Kelvin, and Fahrenheit
To accurately understand the temperature of absolute zero on the Fahrenheit scale, we must first familiarize ourselves with the three major temperature scales: Celsius, Kelvin, and Fahrenheit.
-
Celsius (°C): This scale, widely used internationally, defines the freezing point of water as 0°C and the boiling point as 100°C at standard atmospheric pressure.
-
Kelvin (K): This is the absolute temperature scale. Zero Kelvin (0 K) represents absolute zero. The size of a Kelvin degree is the same as a Celsius degree, meaning a change of 1 K is equivalent to a change of 1 °C.
-
Fahrenheit (°F): This scale is primarily used in the United States. It defines the freezing point of water as 32°F and the boiling point as 212°F at standard atmospheric pressure.
Calculating Absolute Zero on the Fahrenheit Scale
The conversion between Celsius and Kelvin is straightforward: K = °C + 273.15. Therefore, absolute zero (0 K) corresponds to -273.15 °C.
To convert Celsius to Fahrenheit, we use the formula: °F = (°C × 9/5) + 32. Substituting -273.15 °C into this formula, we get:
°F = (-273.15 × 9/5) + 32 = -459.67 °F
Therefore, absolute zero is -459.67°F. This is the lowest possible temperature on the Fahrenheit scale, just as 0 K is the lowest possible temperature on the Kelvin scale.
Implications and Applications of Understanding Absolute Zero
The pursuit of ever-lower temperatures has driven significant technological advancements. Cryogenics, the study of materials at very low temperatures, has led to the development of:
-
Superconducting materials: Many materials exhibit superconductivity at extremely low temperatures, meaning they lose all electrical resistance. This has profound implications for power transmission, magnetic resonance imaging (MRI) technology, and high-speed computing.
-
Advanced medical technologies: Cryogenic techniques are used in various medical procedures, including cryosurgery (tissue freezing) and cryopreservation (tissue storage at very low temperatures).
-
High-precision measurement instruments: Low temperatures are essential for achieving high precision in various scientific instruments, such as those used in astronomy and particle physics.
-
Space exploration: Understanding low-temperature phenomena is crucial for designing spacecraft and equipment that can operate in the extremely cold environments of space.
The Challenges of Reaching Near-Absolute Zero
While we can’t reach absolute zero, achieving temperatures extremely close to it presents significant challenges. These include:
-
Minimizing heat leaks: Even tiny amounts of heat can significantly raise the temperature of a system at extremely low temperatures. This necessitates meticulous insulation and careful experimental design.
-
Dealing with quantum effects: At very low temperatures, quantum mechanical effects become dominant. These effects can influence the behavior of materials in ways that are not readily predictable using classical physics.
-
Developing advanced cooling techniques: Reaching ultra-low temperatures requires sophisticated cooling methods, such as dilution refrigeration or adiabatic demagnetization. These techniques are complex and require specialized equipment.
The Future of Low-Temperature Research
Research continues to push the boundaries of low-temperature physics. Scientists are exploring new materials and techniques to achieve even lower temperatures and to better understand the behavior of matter under these extreme conditions. This research holds the potential for transformative advances in various technological fields, including:
-
Quantum computing: Ultra-low temperatures are crucial for the operation of quantum computers, which rely on the manipulation of quantum states at near absolute zero.
-
New superconducting materials: The search for high-temperature superconductors continues, with the goal of developing materials that exhibit superconductivity at more practical temperatures.
-
Understanding fundamental physics: Research at extremely low temperatures can provide insights into fundamental aspects of physics, such as the nature of dark matter and the properties of quantum entanglement.
Conclusion
Absolute zero, though unattainable, remains a pivotal concept in physics. Understanding its temperature on different scales, such as the Fahrenheit scale (-459.67°F), highlights the fundamental limitations of temperature and provides valuable insights into the behavior of matter at the extreme. The ongoing research in low-temperature physics and cryogenics promises exciting advancements in various technological and scientific domains, furthering our comprehension of the universe and leading to innovative applications that benefit humanity. The quest to approach absolute zero, though challenging, continues to drive scientific curiosity and fuels technological innovation.
Latest Posts
Latest Posts
-
What Is Stationary Phase In Gc
May 10, 2025
-
Which Isotope Is The Most Abundant
May 10, 2025
-
How Do You Find The Area Between Two Z Scores
May 10, 2025
-
How Do You Solve Multi Step Equations With Fractions
May 10, 2025
-
What Is The Function Of The Fruits
May 10, 2025
Related Post
Thank you for visiting our website which covers about Absolute Zero Is What Temperature On The Fahrenheit Scale . We hope the information provided has been useful to you. Feel free to contact us if you have any questions or need further assistance. See you next time and don't miss to bookmark.