A Sequence Of Dna That Codes For A Protein
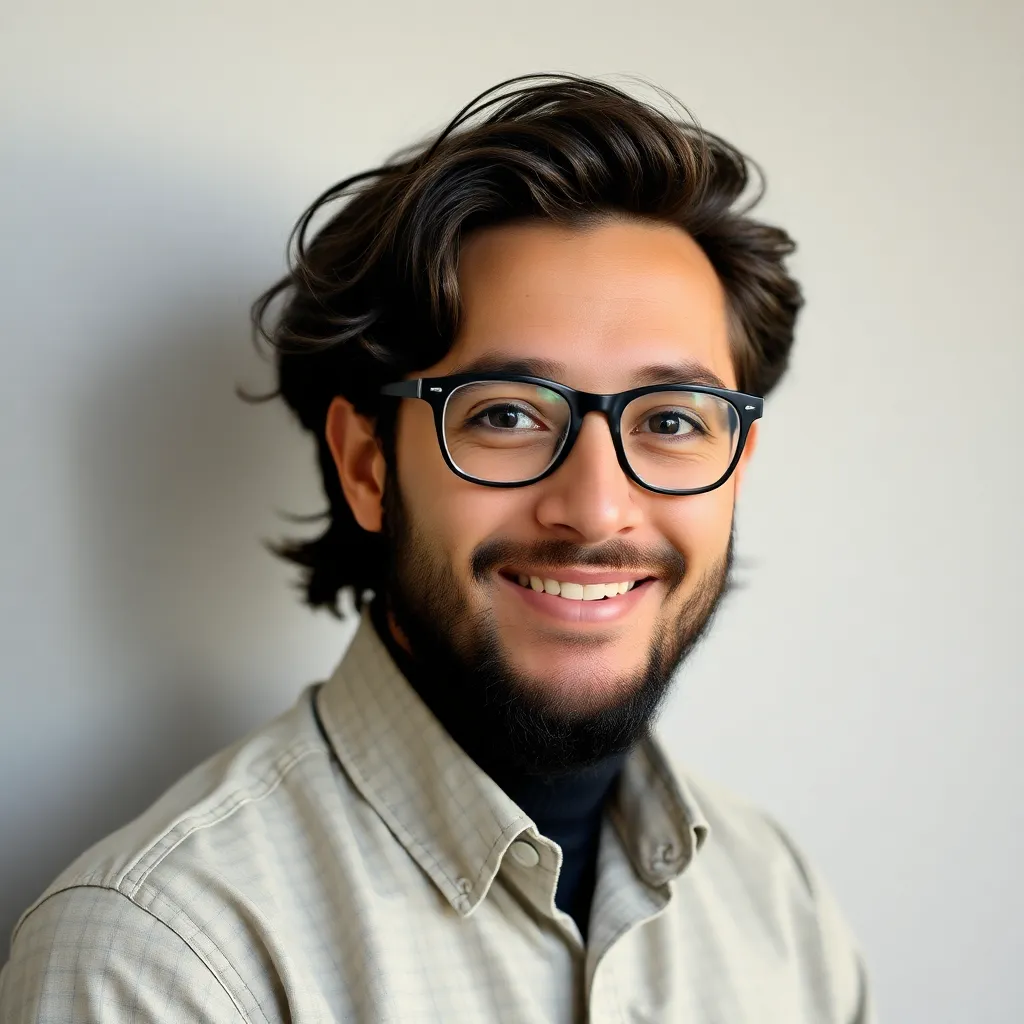
listenit
May 13, 2025 · 7 min read
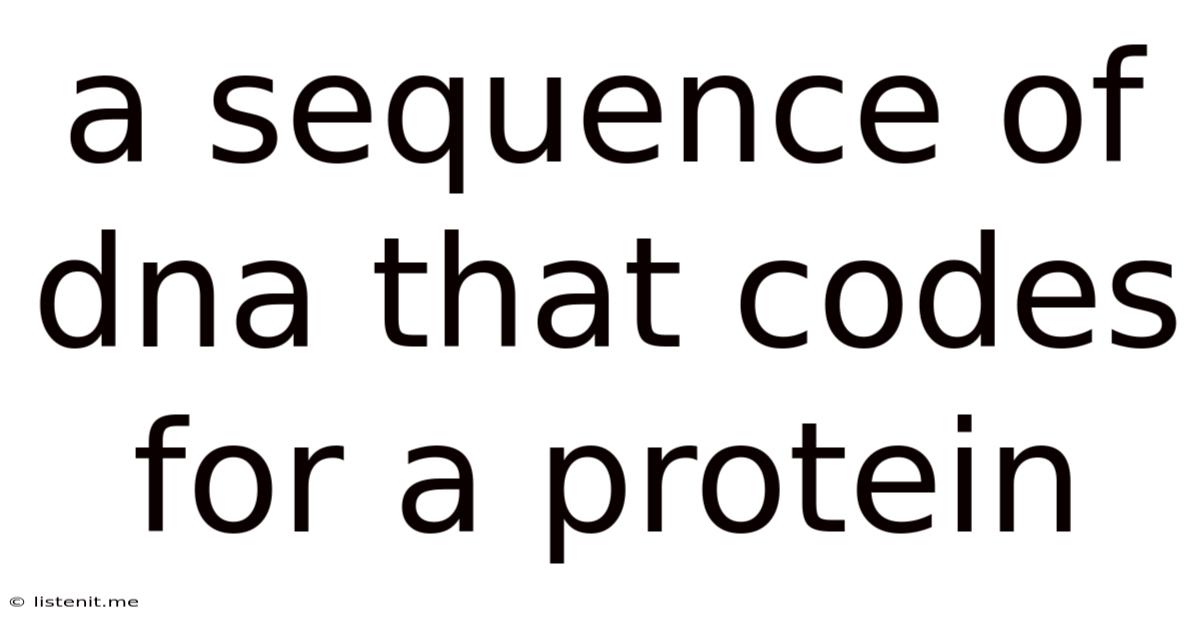
Table of Contents
A Sequence of DNA That Codes for a Protein: Unraveling the Central Dogma of Molecular Biology
The central dogma of molecular biology dictates the flow of genetic information from DNA to RNA to protein. This seemingly simple statement belies an incredibly complex and fascinating process, fundamental to all life on Earth. At the heart of this process lies a specific sequence of DNA – a gene – that acts as the blueprint for a protein. Understanding how this DNA sequence translates into a functional protein is crucial for comprehending a vast array of biological processes, from cell growth and development to disease mechanisms and evolution.
From DNA Sequence to Protein Structure: A Detailed Look
DNA, or deoxyribonucleic acid, is a double-stranded helix composed of nucleotides. Each nucleotide consists of a deoxyribose sugar, a phosphate group, and one of four nitrogenous bases: adenine (A), guanine (G), cytosine (C), and thymine (T). The sequence of these bases along the DNA strand constitutes the genetic code. A gene, the fundamental unit of heredity, is a specific segment of this DNA sequence that carries the instructions for building a single protein.
Transcription: DNA to RNA
The process of protein synthesis begins with transcription, where the DNA sequence of a gene is copied into a messenger RNA (mRNA) molecule. This occurs in the cell's nucleus. The enzyme responsible for this process is RNA polymerase. RNA polymerase binds to a specific region of the DNA called the promoter, unwinds the DNA double helix, and synthesizes a complementary RNA strand. Unlike DNA, RNA contains uracil (U) instead of thymine (T). Thus, an adenine (A) in the DNA template will pair with uracil (U) in the mRNA transcript.
The mRNA molecule, carrying the genetic information from the DNA, then undergoes processing before it can leave the nucleus. This includes:
- Capping: Addition of a modified guanine nucleotide to the 5' end, protecting the mRNA from degradation and aiding in ribosome binding.
- Splicing: Removal of introns (non-coding sequences) and joining of exons (coding sequences). This process is crucial for generating different protein isoforms from a single gene.
- Polyadenylation: Addition of a poly(A) tail to the 3' end, further protecting the mRNA from degradation and aiding in its export from the nucleus.
Translation: RNA to Protein
Once processed, the mature mRNA molecule leaves the nucleus and enters the cytoplasm, where translation occurs. Translation is the process of converting the mRNA sequence into a polypeptide chain, the precursor to a functional protein. This process takes place on ribosomes, complex molecular machines composed of ribosomal RNA (rRNA) and proteins.
The mRNA sequence is read in codons, three-nucleotide sequences that specify a particular amino acid. The genetic code is degenerate, meaning multiple codons can code for the same amino acid. For example, the codons UUU and UUC both code for the amino acid phenylalanine. However, each codon codes for only one amino acid.
Transfer RNA (tRNA) molecules play a crucial role in translation. Each tRNA molecule carries a specific amino acid and recognizes a corresponding codon on the mRNA. The tRNA molecule has an anticodon, a three-nucleotide sequence complementary to the mRNA codon. The ribosome facilitates the binding of the tRNA molecule to the mRNA, ensuring the correct amino acid is added to the growing polypeptide chain.
The ribosome moves along the mRNA, reading each codon sequentially and adding the corresponding amino acid to the polypeptide chain. This process continues until a stop codon is encountered, signaling the termination of translation. The completed polypeptide chain is then released from the ribosome and undergoes folding and modification to become a functional protein.
The Importance of DNA Sequence Accuracy
The accuracy of the DNA sequence is paramount for the correct synthesis of a functional protein. Even a single nucleotide change, or mutation, can have significant consequences. Mutations can result in:
- Missense mutations: A change in a single nucleotide that results in a different amino acid being incorporated into the protein. This can affect the protein's structure and function, sometimes leading to disease.
- Nonsense mutations: A change in a single nucleotide that results in a premature stop codon, leading to a truncated and often non-functional protein.
- Frameshift mutations: Insertions or deletions of nucleotides that are not multiples of three, shifting the reading frame of the mRNA and altering the amino acid sequence downstream of the mutation. This often results in a non-functional protein.
These mutations can be caused by various factors, including errors during DNA replication, exposure to mutagens (e.g., UV radiation, certain chemicals), and transposable elements (jumping genes).
Beyond the Basics: Regulation and Complexity
The process of protein synthesis is not simply a linear progression from DNA to RNA to protein. It is a highly regulated process, subject to a complex interplay of factors that influence gene expression. These factors include:
- Transcriptional regulation: The rate of transcription can be controlled by various factors, including transcription factors that bind to specific DNA sequences near the promoter region, affecting the ability of RNA polymerase to initiate transcription.
- Post-transcriptional regulation: The processing and stability of mRNA molecules can be regulated, influencing the amount of mRNA available for translation.
- Translational regulation: The rate of translation can be controlled by various factors, including the availability of ribosomes, tRNA molecules, and initiation factors.
- Post-translational modification: After translation, proteins can undergo a variety of modifications, such as glycosylation, phosphorylation, and ubiquitination, that alter their structure, function, and stability.
The Role of DNA Sequence in Disease
Many diseases are caused by mutations in DNA sequences that alter the structure or function of proteins. Examples include:
- Sickle cell anemia: A single nucleotide change in the gene encoding beta-globin results in a missense mutation, causing the production of abnormal hemoglobin that leads to the characteristic sickle shape of red blood cells.
- Cystic fibrosis: Mutations in the CFTR gene, which encodes a chloride channel protein, cause defects in chloride transport across cell membranes, leading to the accumulation of thick mucus in the lungs and other organs.
- Huntington's disease: An expansion of a trinucleotide repeat in the huntingtin gene leads to the production of a protein with an elongated polyglutamine tract, causing neurodegeneration.
Understanding the relationship between DNA sequence, protein structure, and function is crucial for developing diagnostic tools and therapies for these and other genetic diseases.
Applications and Future Directions
The ability to determine the DNA sequence of genes has revolutionized biology and medicine. This knowledge has led to the development of numerous applications, including:
- Genetic testing: Identifying individuals at risk for genetic diseases.
- Pharmacogenomics: Developing personalized medicine tailored to an individual's genetic makeup.
- Gene therapy: Developing treatments for genetic diseases by correcting faulty genes or introducing new genes.
- Biotechnology: Engineering proteins with novel properties for industrial and medical applications.
The field continues to evolve rapidly, with ongoing research focused on understanding the complex interplay of genes and the environment, developing more sophisticated gene editing technologies, and unraveling the intricacies of the human genome. This research holds immense promise for addressing major health challenges and improving human health.
Conclusion: The Ongoing Story of the Gene
The DNA sequence that codes for a protein is a fundamental element of life, a testament to the elegance and complexity of biological systems. From the intricacies of transcription and translation to the impact of mutations and the regulation of gene expression, understanding this process is crucial for advancing our knowledge of biology and medicine. As technology continues to advance, our ability to manipulate and understand these sequences will continue to unlock new possibilities for treating diseases, developing new technologies, and pushing the boundaries of our understanding of the living world. The ongoing exploration of the gene, the basic unit of heredity, remains a vibrant and essential field of scientific inquiry.
Latest Posts
Latest Posts
-
Why Do Electric Field Lines Never Cross
May 13, 2025
-
1 10 As A Percent And Decimal
May 13, 2025
-
Can All Minerals Be A Gemstone
May 13, 2025
-
Multicellular Heterotrophs Without A Cell Wall
May 13, 2025
-
What Are The Gcf Of 48
May 13, 2025
Related Post
Thank you for visiting our website which covers about A Sequence Of Dna That Codes For A Protein . We hope the information provided has been useful to you. Feel free to contact us if you have any questions or need further assistance. See you next time and don't miss to bookmark.